ABSTRACT
Objective: The aim of this study was to identify the best experimental model in which to observe the pulmonary alterations characterizing
hepatopulmonary syndrome (HPS). Methods: Male Wistar rats, with mean weight of 250 g, were used in four experimental models: inhaled
carbon tetrachloride; intraperitoneal carbon tetrachloride; partial portal vein ligation; and bile duct ligation (BDL). The animals in all groups were
divided into control and experimental subgroups. The following variables were measured: transaminase levels; blood gases; lipoperoxidation,
using thiobarbituric acid reactive substances (TBARS) and chemiluminescence; and levels of superoxide dismutase (SOD) anti-oxidant activity.
Anatomopathological examination of the lung was also performed. Results: There were statistically significant differences between the BDL
control and BDL experimental groups: aspartate aminotransferase (105.3 ± 43 vs. 500.5 ± 90.3 IU/L); alanine aminotransferase (78.75 ± 37.7 vs.
162.75 ± 35.4 IU/L); alkaline phosphatase (160 ± 20.45 vs. 373.25 ± 45.44 IU/L); arterial oxygen tension (85.25 ± 8.1 vs. 49.9 ± 22.5 mmHg);
and oxygen saturation (95 ± 0.7 vs. 73.3 ± 12.07%). Lipoperoxidation and antioxidant activity also differed significantly between the two
BDL groups (control vs. experimental): TBARS (0.87 ± 0.3 vs. 2.01 ± 0.9 nmol/mg protein); chemiluminescence (16008.41 ± 1171.45 vs.
20250.36 ± 827.82 cps/mg protein); and SOD (6.66 ± 1.34 vs. 16.06 ± 2.67 IU/mg protein). The anatomopathological examination confirmed
pulmonary vasodilatation in the BDL model. In the other models, there were no alterations that were characteristic of HPS. Conclusions: The
data obtained suggest that the BDL model can be used in future studies involving hepatic alterations related to oxidative stress and HPS.
Keywords:
Hepatopulmonary syndrome; Lung; Oxidative stress; Rats.
RESUMO
Objetivo: O objetivo deste trabalho foi avaliar o melhor modelo experimental para observar alterações pulmonares que caracterizam a
síndrome hepatopulmonar (SHP). Métodos: Ratos machos Wistar, com peso médio de 250 g foram usados em quatro modelos experimentais:
tetracloreto de carbono inalatório; tetracloreto de carbono intraperitoneal; ligadura parcial de veia porta; e ligadura de ducto biliar (LDB). Em
todos os grupos os animais foram divididos em controle e experimental. Foram avaliadas as seguintes variáveis: transaminases; gasometria;
lipoperoxidação por substâncias que reagem ao ácido tiobarbitúrico (TBARS) e por quimiluminescência; e atividade antioxidante da enzima
superóxido dismutase (SOD). Foi feito também o exame anatomopatológico do pulmão. Resultados: Observou-se diferenças significativas
entre os grupos LDB controle e experimental: aspartato amino transferase (105,3 ± 43 vs. 500,5 ± 90,3 UI/L); alanino aminotransferase
(78,75 ± 37,7 vs. 162,75 ± 35,4 UI/L); fosfatase alcalina (160 ± 20,45 vs. 373,25 ± 45,44 UI/L); pressão parcial de oxigênio (85,25 ± 8,1 vs.
49,9 ± 22,5 mmHg); e saturação de hemoglobina (95 ± 0,7 vs. 73,3 ± 12,07%). A lipoperoxidação e a atividade antioxidante também
demonstrou diferenças entre os dois grupos LDB (controle vs. experimental): TBARS (0,87 ± 0,3 vs. 2,01 ± 0,9 nmol/mg proteína); quimiluminescência
(16008,41 ± 1171,45 vs. 20250,36 ± 827,82 cps/mg proteína); e SOD (6,66 ± 1,34 vs. 16,06 ± 2,67 UI/mg proteína). No exame
anatomopatológico observou-se vasodilatação pulmonar no modelo de LDB. Conclusões: Os dados sugerem que o modelo de LDB pode ser
usado para outros estudos envolvendo alterações hepáticas e suas relações com o estresse oxidativo e a SHP.
Palavras-chave:
Síndrome hepatopulmonar; Pulmão; Estresse oxidativo; Ratos.
IntroductionLiver cirrhosis is a chronic progressive disease that represents an irreversible or slowly reversible stage of hepatic dysfunction, characterized by the formation of fibrotic nodules. It occurs as a result of cicatrization and hepatocellular regeneration, which constitute the main responses of the liver tissue to countless inflammatory, toxic, metabolic and congestive insults.(1)
Abnormalities of arterial oxygenation are common in patients with cirrhosis, a great difference in the alveolar-arterial oxygen gradient and severe hypoxemia being observed in 3-7% of cases (arterial oxygen tension-PaO2-below 60 mmHg).(2) The physiopathology of pulmonary dysfunction in the presence of liver diseases might involve a decrease in the attenuation of hypoxic vasoconstriction, low vascular resistance, ventilation-perfusion imbalance, intrapulmonary shunt and alteration in gas diffusion. These clinical and laboratory findings characterize hepatopulmonary syndrome (HPS), which is seen in patients with cirrhosis or portal hypertension and consists of cirrhosis accompanied by hypoxemia and intrapulmonary vasodilatation, although without cardiopulmonary disease.(3)
Experimental induction of liver disease can accurately replicate the histological, biochemical, hemodynamic, renal and neurohumoral alterations seen in human liver dysfunction. Handling risks to the researcher have been eliminated, and the use of such models improves our understanding of the physiopathology of liver disease and of other syndromes involved. We can follow the physiopathological progress of cirrhosis and its complications through experimental studies in models of liver cirrhosis, secondary biliary cirrhosis and portal hypertension. In such models, liver cirrhosis is induced by administration of carcinogenic agents such as carbon tetrachloride (CCl4), biliary cirrhosis is induced through bile duct ligation (BDL), and portal hypertension is induced by partial portal vein ligation (PPVL).(4) Among the numerous complications of HPS, some of the most serious are the pulmonary alterations, which are primarily characterized by low oxygen concentrations and intrapulmonary vasodilatation. To date, there is no specific model to study such HPS-related alterations. Therefore, the aim of this study was to compare four experimental models in terms of their ability to demonstrate the pulmonary complications occurring in HPS. To that end, we analyzed liver function, blood gas exchange, anatomopathological aspects and oxidative stress (to quantify tissue damage).
MethodsFor the purposes of this study, 40 male Wistar rats (mean body weight, 250 g) were obtained from the Universidade Federal do Rio Grande do Sul (UFRGS, Federal University of Rio Grande do Sul) Laboratory Animal Breeding Facility. The rats were housed in plastic cages (47 × 34 × 18 cm) lined with woodchips and were maintained on a 12/12-h light/dark cycle in a temperature-controlled environment (20-25°C) with ad libitum access to standard rat chow and water. All experimental procedures complied with the guidelines established by the Porto Alegre Hospital de Clínicas ethics committees: the Research in Health and Animal Rights Committee; and the Research and Postgraduate Group Committee on Research and
Ethics in Health. Four experimental models were evaluated:
1) Inhaled CCl4 (IN CCl4) to induce cirrhosis: Animals were divided into two subgroups: control (IN CCl4-Co, n = 5), receiving only phenobarbital in drinking water; and experimental (IN CCl4-Ex, n = 5), receiving phenobarbital in drinking water plus IN CCl4. Phenobarbital was added to the drinking water at a concentration of 0.3 g/L in order to evoke enzymatic induction and accelerate the cirrhotic process. The IN CCl4-Ex group was exposed to the aggressive agent twice a week (on Mondays and Fridays), according to the protocol devised by Jiménez et al.,(4) in an inhalation chamber (47 × 34 × 18 cm). The CCl4 was placed in glass container (humidifier) attached to an air compressor and released into the chamber at a flow rate of 1 L/min. In the first three sessions, the duration of exposure was 30 sec and the animals remained within the chamber for an additional 30 sec while the compressor was off (waiting time). In the fourth session, the duration of exposure was increased to 60 sec, followed by an additional 60 sec in waiting mode. Subsequently, the duration of exposure and the waiting time were increased by 30 sec every three sessions, reaching a maximum of 5 min at week 16.(4,5)
2) Intraperitoneal CCl4 (IP CCl4) to induce cirrhosis: Animals were divided into two subgroups: control (IP CCl4-Co, n = 5), receiving only phenobarbital in drinking water; and experimental (IP CCl4-Ex, n = 5), receiving phenobarbital in drinking water plus IP CCl4. Phenobarbital was given as described above. Cirrhosis was induced by IP injection of CCl4 (0.5 mL, diluted 1:6 in vegetable oil, at 10:00 p.m. every 4 days for 14 weeks).(6)
3) PPVL to induce portal hypertension: We used the model of PPVL described in a study published in 1983.(7) Animals were divided into two subgroups: control (PPVL-Co, n = 5), submitted to sham partial portal vein ligation; and experimental (PPVL-Ex, n = 5), submitted to ligation. In brief, the animals were anesthetized and submitted to mid-ventral laparotomy and the portal vein was isolated using a 20-gauge needle, gently withdrawing the needle after the ligation. Upon completion of the procedure, the peritoneum and abdominal wall were sutured.
4) BDL to induce biliary cirrhosis: We followed the model of secondary biliary cirrhosis secondary to BDL described in a study published in 1985.(8) Animals were divided into two subgroups: control (BDL-Co, n = 5), submitted to sham common BDL; and experimental (BDL-Ex, n = 5), submitted to BDL.
Laboratory tests were performed at the Central Laboratory of the Santa Casa Hospital Complex in Porto Alegre, and all other procedures were performed at the Laboratory of Physiology, Hepatology and Oxidative Stress of the UFRGS Porto Alegre Hospital de Clínicas.
Prior to all surgical procedures, animals were anesthetized with 2% xylazine hydrochloride (50 mg/kg body weight) and ketamine hydrochloride (100 mg/kg body weight), both administered intraperitoneally. Following the experimental procedures, the animals were housed in individual cages for a period of two weeks, at which time they were killed with an overdose of those same anesthetic agents.
On the day of sacrifice, the animals were anesthetized and blood samples were collected from the retro-ocular sinus.(9) Those blood samples were used in order to assess the serum levels of aspartate aminotransferase (AST), alanine aminotransferase (ALT) and alkaline phosphatase (ALP), which are expressed as IU/L. Subsequently, the abdominal region was shaved, a mid-ventral laparotomy was performed, and blood was collected from the abdominal aorta artery in order to measure arterial blood gas exchange with an ABL 700 analyzer (Radiometer, Copenhagen, Denmark), using the iontophoresis method to determine PaO2, arterial carbon dioxide tension (PaCO2), and arterial oxygen saturation (SaO2).
After the blood samples had been collected, the animals were killed as described above. The lungs were then dissected out and weighed in order to determine the relationship between the weight of the lung and the total body weight (the so-called lung weight/body weight ratio). The anterior part of the right inferior lobe was then set aside for the histological analysis, the remainder promptly being frozen in liquid nitrogen and stored at −80 °C for later analysis. The frozen tissue was homogenized in phosphate buffer (KCl 140 mM, phosphate 20 mM, pH 7.4) using an Ultra-Turrax homogenizer (IKA Labortechnik, Staufen, Germany) and centrifuged at 3000 rpm for 10 min. Lipoperoxidation was determined through determination of the levels of thiobarbituric acid reactive substances (TBARS)(10) and chemiluminescence.(11) Activity of the antioxidant enzyme superoxide dismutase (SOD) was determined by quantifying the oxidation of adrenaline to adrenochrome.(12)
The samples for histological analysis of the pulmonary tissue were collected and stored for 12 h in 10% formaldehyde solution, then transferred to 70% alcohol and stained with hematoxylin-eosin. The anatomopathological examination was performed in double-blind fashion by a pathologist in the Pathology Laboratory of the Porto Alegre Hospital de Clínicas.
Means and standard errors of the means were calculated. Data were analyzed using analysis of variance. Post hoc comparisons were carried out using the Student Newman-Keuls test. The level of statistical significance was set at p < 0.05. The Statistical Package for the Social Sciences, version 13.0 (SPSS Inc., Chicago, IL, USA) was used.
ResultsAs can be seen in Table 1, there was a significant difference between the IN CCl4-Co and IN CCl4-Ex groups in terms of the serum levels of ALT and ALP (higher in the IN CCl4-Ex group; p < 0.05 for both), between the IP CCl4-Co and IP CCl4-Ex groups in terms of the serum levels of AST and ALT (higher in the IP CCl4-Ex group; p < 0.05 for both) and between the BDL-Co and BDL-Ex groups in terms of the serum levels of AST and ALT (higher in the BDL-Ex group; p < 0.05 for both). No significant differences were found between the PPVL-Co and PPVL-Ex groups in terms of serum levels of AST, ALT or ALP.
In order to ascertain alterations in gas exchanges, we performed blood gas analysis (Table 1), in which PaO2, PaCO2, SaO2 and the alveolar-arterial oxygen gradient were analyzed in the different models and groups. There was a significant difference between the IN CCl4-Co and IN CCl4-Ex groups in terms of PaO2 (p < 0.001). In the IP CCl4 and PPVL models, there were no significant differences between the control and experimental groups in terms of any blood gas analysis parameters. However, there were significant differences between the BDL-Co and BDL-Ex groups for PaO2, PaCO2 and SaO2 (p < 0.05 for all), as well as for the alveolar-arterial oxygen gradient (p < 0.001).
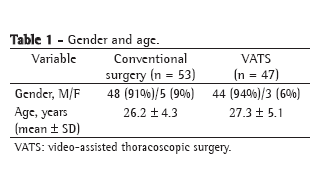
As shown in Table 2, there were significant differences between the control and experimental groups in the IN CCl4 and BDL models in terms of the lung weight/body weight ratio (p < 0.05 in both models), although no such differences were seen in the IP CCl4 or PPVL models.
Table 3 shows lipoperoxidation, characterized by TBARS values, chemiluminescence and SOD activity. In the IN CCl4, IP CCl4 and PPVL models, there were no significant differences between the control and experimental groups. However, there was significantly greater lipoperoxidation in the BDL-Ex group than in the BDL-Co group (p < 0.05).
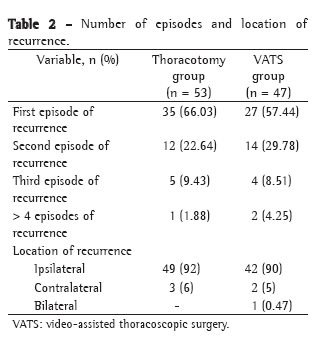
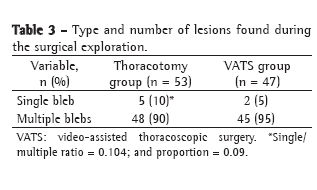
Pulmonary SOD activity was significantly greater in the IN CCl4-Ex, IP CCl4-Ex and BDL-Ex groups than in their corresponding control groups (p < 0.05), although there was no difference between the PPVL-Ex group and the PPVL-Co group.
As can be seen in Figure 1, the histological analysis of lung tissues from animals in all control groups showed normal architecture of the pulmonary parenchyma and vessels with normal diameters. Only one lung tissue sample from a control group animal is shown, since the histological findings were similar for all control group animals. Vessel diameters were also normal in the lungs of experimental group animals of the IN CCl4, IP CCl4 and PPVL models, although greater diameters were observed in the experimental group animals of the BDL model.
DiscussionIn this study, four experimental models were used, three involving induction of cirrhosis and one involving induction of portal hypertension. The CCl4 is used as an inducer of cirrhosis, accurately replicating hemodynamic abnormalities, with increased hepatic vascular resistance, leading to portal hypertension and hindering exchanges between the sinusoids and the hepatocytes, all of which are responsible for the greatest hepatic damage in cirrhosis.(13,14) The IN CCl4 model combines CCl4 inhalation with the administration of phenobarbital in drinking water, which accelerates the metabolic process by increasing the activity of the cytochrome P450 system, thereby leading to the development of cirrhosis, with splenomegaly and pronounced ascites, the latter being a prominent feature of chronic liver failure during cirrhosis.(6,15) In the present study, the serum levels of AST, ALT and ALP were higher in the experimental group animals than in their respective control groups for both CCl4 models, indicating a loss of hepatic integrity in the IN CCl4-Ex and IP CCl4-Ex animals, since elevated serum levels of these enzymes are directly related to cell damage and active necrosis of liver cells.
Patients with portal hypertension develop increased blood flow to the stomach. The physiological findings that correlate with worsening portal hypertensive gastropathy include increased portal venous pressure gradient and decreased hepatic blood flow. At a prevalence of 80% of cases, portal hypertension is directly related to the duration of liver injury and the extent of gastropathy.(16) In the present study, serum levels of AST, ALT and ALP were normal in the PPVL-Ex and PPVL-Co group animals, indicating a lack of hepatic alterations. This can be explained by the fact that PPVL is a model of portal hypertension, which can be a complication of liver cirrhosis, rather than a model of cirrhosis itself. These findings corroborate those of a previous study involving an animal model of portal hypertension.(17) Liver cirrhosis was observed in the experimental group animals of all models, with the exception of the PPVL model.
We found serum levels of ALT, AST and ALP to be higher in the BDL-Ex group than in the BDL-Co group. In the majority of patients with secondary biliary cirrhosis, the hepatobiliary damage resulting from biliary obstruction produces hyperbilirubinemia, higher ALP and higher aminotransferase levels. The elevated levels of these enzymes are largely attributable to necrosis of aminotransferase-rich tissues, as well as to the cholestasis provoked in this model.(18) Our findings demonstrate the hepatic impairment produced in animals submitted to this model.
In the BDL model, lung weight/body weight ratio were greater in the BDL-
Ex group than in the BDL-Co group. One animal study showed a post-BDL increase in arteriole diameters.(19) In the present study, the difference in lung weight/body weight ratio was probably due to the shunt and intrapulmonary vascular dilatation caused by the accumulation of blood in the organ.
The evaluation of arterial blood gases is an indicator used to facilitate the diagnosis of HPS. In most cirrhotic patients, PaO2 is < 80 mmHg, SaO2 is ≤92%, and PaCO2 is ≈30 mmHg.(20) In our study, we demonstrated significant differences between the experimental and control groups in terms of these parameters. Experimental studies have shown that control animals present PaO2 values of 90-99 mmHg and PaCO2 values of ≈40 mmHg,(21,22) values comparable to those seen in the IN CCl4-Co group animals evaluated in the present study. We observed that PaO2 and SaO2 were significantly lower the IN CCl4-Ex group than in the IN CCl4-Co group, whereas PaCO2 was higher, although not significantly so. These alterations are probably due to the toxic potential of inhaled CCl4 in the respiratory system. The PPVL model has not been associated with abnormalities in the diffusion of pulmonary gases,(23) as demonstrated in our study. We observed significant differences between the BDL-Ex and BDL-Co groups in terms of the blood gas analysis findings, demonstrating that animals with secondary biliary cirrhosis present hypoxemia. This hypoxemia might be associated with the bacterial translocation that BDL causes in 45-75% of cirrhotic animals, in which the BDL-induced hepatocellular lesion hinders adequate blood filtration, promoting the development of portosystemic shunts and a dramatic decrease of the phagocytic capacity of the liver, allowing bacteria and endotoxins to enter the pulmonary circulation. In this situation, the lung filters the blood, compensating for the decreased phagocytic function of the liver. During phagocytosis, the activation of numerous macrophages results in the secretion of products such as cytokines and nitric oxide into the extracellular medium, nitric oxide being a potent vasodilator and also being involved in mechanisms of imbalance between the antioxidant and oxidative systems.(24) Nitric oxide functions as a molecular signaler (mediating vasodilatation when produced at low concentrations by nitric oxide synthase in endothelial vascular cells) and as a highly toxic oxidant source (when produced at high concentration by nitric oxide synthase in macrophages in the inflammatory process), thereby promoting intrapulmonary vasodilatation followed by hypoxemia.(25)
Many authors have reported that, in the presence of cirrhosis, there is a considerable increase of lipoperoxidation due to the formation of reactive oxygen species.(26) In experimental models, this phenomenon also occurs, leading to morphological alterations involving the endoplasmic reticulum of hepatocytes. In the CCl4 model, these alterations derive from the metabolism of the toxic agent CCl4 itself, which is metabolized into the free radicals trichloromethyl and trichloromethyl peroxyl, injurious radicals that can initiate the lipoperoxidation process.(27)
Although hepatic peroxidation has been widely described in models of CCl4-induced cirrhosis as well as in models of PPVL-induced portal hypertension, the same has not been observed in pulmonary tissue.(6,15,17) Nevertheless, in the present study, we found that SOD activity was altered in the homogenates of pulmonary tissue samples collected from animals in the in the IN CCl4-Ex and BDL-Ex groups.
In the IN CCl4-induced cirrhosis model, alterations in blood gas exchange and elevated levels of SOD activity are observed, possibly as a defense against the oxidative damage brought about by CCl4 inhalation. However, these alterations are not characteristic of HPS, since inhaled CCl4 has toxic effects on the lungs and kidneys that are compounded by the high arterial concentrations of the drug, which promote its passage through the lungs, through the pulmonary circulation and into the left atrium.(28)
In the present study, the animals submitted to BDL presented significantly greater lipoperoxidation (higher levels of TBARS and chemiluminescence) than did the corresponding controls. This finding might be associated with increased production of free radicals, since bacterial translocation and phagocytic migration into the pulmonary tissue increase the generation of superoxide anions capable of oxidizing the plasmatic membrane, generating a great quantity of reactive oxygen species. It is possible that, in response to this damage, there was an increase in the activity of endogenous SOD, which plays an important role in the cell redox balance, since it dismutates the generated free radicals in an attempt to protect the tissue against injury.
Among the various classical models of experimental cirrhosis and portal hypertension, the model of BDL-induced cirrhosis appears to be the most useful for the study of HPS, since it produces true cirrhosis and alters all arterial gas exchange parameters, as well as increasing lipoperoxidation and SOD antioxidant defense.
References 1. Onori P, Morini S, Franchitto A, Sferra R, Alvaro D, Gaudio E. Hepatic microvascular features in experimental cirrhosis: a structural and morphometrical study in CCl4-treated rats. J Hepatol. 2000;33(4):555-63.
2. Rodriguez-Roisin R, Agusti A, Roca J. Pulmonary function and liver disease. Current Opinion Gastroenterol. 1988;4:609-14.
3. Rodríguez-Roisin R, Agustí AG, Roca J. The hepatopulmonary syndrome: new name, old complexities. Thorax. 1992;47(11):897-902.
4. Jiménez W, Clária J, Arroyo V, Rodés J. Carbon tetrachloride induced cirrhosis in rats: a useful tool for investigating the pathogenesis of ascites in chronic liver disease. J Gastroenterol Hepatol. 1992;7(1):90-7.
5. McLean EK, McLean AE, Sutton PM. Instant cirrhosis. An improved method for producing cirrhosis of the liver in rats by simultaneous administration of carbon tetrachloride and phenobarbitone. Br J Exp Pathol. 1969;50(5):502-6.
6. Pavanato A, Tuñón MJ, Sánchez-Campos S, Marroni CA, Llesuy S, González-Gallego J, et al. Effects of quercetin on liver damage in rats with carbon tetrachloride-induced cirrhosis. Dig Dis Sci. 2003;48(4):824-9.
7. Vorobioff J, Bredfeldt JE, Groszmann RJ. Hyperdynamic circulation in portal-hypertensive rat model: a primary factor for maintenance of chronic portal hypertension. Am J Physiol. 1983;244(1):G52-7.
8. Kountouras J, Billing BH, Scheuer PJ. Prolonged bile duct obstruction: a new experimental model for cirrhosis in the rat. Br J Exp Pathol. 1984;65(3):305-11.
9. Halpern BN, Pacaud A. [Technique of obtaining blood samples from small laboratory animals by puncture of ophthalmic plexus.][Article in Undetermined Language]. C R Seances Soc Biol Fil. 1951;145(19-20):1465-6.
10. Buege JA, Aust SD. Microsomal lipid peroxidation. Methods Enzymol. 1978;52:302-10.
11. Gonzalez Flecha B, Llesuy S, Boveris A. Hydroperoxide-initiated chemiluminescence: an assay for oxidative stress in biopsies of heart, liver, and muscle. Free Radic Biol Med. 1991;10(2):93-100.
12. Misra HP, Fridovich I. The role of superoxide anion in the autoxidation of epinephrine and a simple assay for superoxide dismutase. J Biol Chem. 1972;247(10):3170-5.
13. Hernández-Muñoz R, Díaz-Muñoz M, López V, López-Barrera F, Yáñez L, Vidrio S, et al. Balance between oxidative damage and proliferative potential in an experimental rat model of CCl4-induced cirrhosis: protective role of adenosine administration. Hepatology. 1997;26(5):1100-10.
14. Corrales F, Giménez A, Alvarez L, Caballería J, Pajares MA, Andreu H, et al. S-adenosylmethionine treatment prevents carbon tetrachloride-induced S-adenosylmethionine synthetase inactivation and attenuates liver injury. Hepatology. 1992;16(4):1022-7.
15. Cremonese RV, Pereira-Filho AA, Magalhães R, de Mattos AA, Marroni CA, Zettler CG, et al. [Experimental cirrhosis induced by carbon tetrachloride inhalation: adaptation of the technique and evaluation of lipid peroxidation][Article in Portuguese]. Arq Gastroenterol. 2001;38(1):40-7.
16. Primignani M, Carpinelli L, Preatoni P, Battaglia G, Carta A, Prada A, et al. Natural history of portal hypertensive gastropathy in patients with liver cirrhosis. The New Italian Endoscopic Club for the study and treatment of esophageal varices (NIEC). Gastroenterology. 2000;119(1):181-7.
17. Moreira AJ, Fraga C, Alonso M, Collado PS, Zetller C, Marroni C, et al. Quercetin prevents oxidative stress and NF-kappaB activation in gastric mucosa of portal hypertensive rats. Biochem Pharmacol. 2004;68(10):1939-46.
18. Pastor A, Collado PS, Almar M, González-Gallego J. Antioxidant enzyme status in biliary obstructed rats: effects of N-acetylcysteine. J Hep atol. 1997;27(2):363-70.
19. Berthelot P, Walker JG, Sherlock S, Reid L. Arterial changes in the lungs in cirrhosis of the liver--lung spider nevi. N Engl J Med. 1966;274(6):291-8.
20. Krowka MJ, Cortese DA. Hepatopulmonary syndrome: an evolving perspective in the era of liver transplantation. Hepatology. 1990;11(1):138-42.
21. Zhang XJ, Katsuta Y, Akimoto T, Ohsuga M, Aramaki T, Takano T. Intrapulmonary vascular dilatation and nitric oxide in hypoxemic rats with chronic bile duct ligation. J Hepatol. 2003;39(5):724-30.
22. Zhang HY, Han DW, Wang XG, Zhao YC, Zhou X, Zhao HZ. Experimental study on the role of endotoxin in the development of hepatopulmonary syndrome. World J Gastroenterol. 2005;11(4):567-72.
23. Fallon MB. Mechanisms of pulmonary vascular complications of liver disease: hepatopulmonary syndrome. J Clin Gastroenterol. 2005;39(4 Suppl 2):S138-S42.
24. Rabiller A, Nunes H, Lebrec D, Tazi KA, Wartski M, Dulmet E, et al. Prevention of gram-negative translocation reduces the severity of hepatopulmonary syndrome. Am J Respir Crit Care Med. 2002;166(4):514-7.
25. Thannickal VJ, Fanburg BL. Reactive oxygen species in cell signaling. Am J Physiol Lung Cell Mol Physiol. 2000;279(6):L1005-28.
26. Britton RS, Bacon BR. Role of free radicals in liver diseases and hepatic fibrosis. Hepatogastroenterology. 1994;41(4):343-8.
27. Rechnagel RO, Glende EA Jr. Carbon tetrachloride hepatotoxicity: an example of lethal cleavage. CRC Crit Rev Toxicol. 1973;2(3):263-97.
28. Proctor E, Chatamra K. High yield micronodular cirrhosis in the rat. Gastroenterology. 1982;83(6):1183-90.
___________________________________________________________________________________________________________________
Study conducted at the Hospital de Clinicas de Porto Alegre, Porto Alegre, Brasil.
1. Doctoral Student in the Post-Graduate Program of Physiology at the Universidade Federal do Rio Grande do Sul - UFRGS, Federal University of Rio Grande do Sul - Porto Alegre, Brazil.
2. Doctoral Student in the Post-Graduate Program of Medical Sciences at the Universidade Federal do Rio Grande do Sul − UFRGS, Federal University of Rio Grande do Sul − Porto Alegre, Brazil.
3. Coordinator of the Professional Masters Program in Rehabilitation and Inclusion at the Centro Universitário Metodista IPA − IPA Methodist University Center − Porto Alegre, Brazil.
4. Associate Professor of Gastroenterology,Universidade Federal de Ciências da Saúde de Porto Alegre, Porto Alegre, Brazil.
5. Researcher in the Laboratory of Hepatology and Experimental Physiology at the Hospital de Clinicas de Porto Alegre-Universidade Luterana do Brasil − HCPAULBRA, Lutheran University of Brazil-Hospital de Clinicas de Porto Alegre − Porto Alegre, Brazil.
Correspondence to: Norma Possa Marroni. Rua José Kanan Aranha, 102, Jardim Isabel, CEP 91760-470, Porto Alegre (RS) Brasil.
Tel 55 51 32483202. Fax 55 51 32483202. E-mail: nmarroni@terra.com.br
Apoio Financeiro: This study received financial support in the form of grants from the Coordenação de Aperfeiçoamento de Pessoal de Nível Superior (CAPES), Fundo de Incentivo à Pesquisa e Eventos do Hospital de Clínicas de Porto Alegre (FIPE-HCPA).
Submitted: 20 June 2007. Accepted, after review: 3 October 2007.
**A versão completa em português deste artigo está disponível em www.jornaldepneumologia.com.br