ABSTRACT
Knowledge of the structure and function of pulmonary circulation has evolved considerably in the last few decades. The use of non-invasive imaging techniques to assess the anatomy and function of the pulmonary vessels and heart has taken on added importance with the recent advent of novel therapies. Imaging findings not only constitute a diagnostic tool but have also proven to be essential for prognosis and treatment follow-up. This article reviews the myriad of imaging methods currently available for the assessment of pulmonary circulation, from the simple chest X-ray to techniques that are more complex and promising, such as electrical impedance tomography.
Keywords:
Pulmonary circulation; Diagnostic imaging; Hypertension, pulmonary.
RESUMO
O conhecimento sobre a estrutura e a função da circulação pulmonar evoluiu sensivelmente nas últimas décadas. A utilização de exames de imagem não invasivos para a avaliação da anatomia e da função dos vasos pulmonares e do coração ganhou ainda mais importância com o advento de tratamentos até então indisponíveis. Além do auxílio para o diagnóstico, as informações obtidas têm se mostrado fundamentais para o estabelecimento de prognósticos e como parâmetro de sucesso dos tratamentos. A presente revisão discute os diversos métodos que podem ser utilizados para a avaliação da circulação pulmonar por imagens existentes nos dias de hoje, desde técnicas amplamente disponíveis e de relativa baixa complexidade técnica, como a radiografia de tórax, até métodos complexos e promissores, como a tomografia de impedância elétrica.
Palavras-chave:
Circulação pulmonar; Diagnóstico por imagem; Hipertensão pulmonar.
IntroductionThe physiology of the pulmonary circulation has only recently come to be understood. That is because the techniques available at the beginning of the 20th century did not allow direct assessment of the pulmonary vasculature. Until the late 1930s, there were no methods capable of providing information about cardiopulmonary interaction or the ventilation/perfusion ratio. The milestone breakthrough came in 1940, when the integration of the physiological studies by Cournard et al.(1) and Forssmann's self-experimentation in 1929,(2) which consisted of radiographic documentation of the presence of a catheter in his own heart, culminated in catheterization of the right ventricle (RV) and pulmonary arteries, thereby revolutionizing knowledge in the area of cardiopulmonary medicine. Access to hemodynamic pressure and flow measurements, as well as to blood gas measurements, provided a unique opportunity for advances in the assessment of pulmonary circulation, which became recognized as an exclusively arterial system, characterized by high compliance and low resistance, capable of accommodating large blood volumes and high blood flows at low pressures.(3)
Invasive hemodynamic assessment has become a valuable imaging tool, because it has clarified not only the physiological aspect of the pulmonary circulation but also the anatomical distribution of the pulmonary vessels and features of the right heart.
After the anatomical and functional status of the pulmonary circulation had been addressed, right heart catheterization came to be widely used for the assessment of the pulmonary vasculature, especially in the presence of disease, such as pulmonary hypertension (PH). However, despite being considered the gold standard, the method has major limitations by current standards, because it is invasive in nature, costly, and involves the use of radiation. However, the push to develop noninvasive techniques for monitoring pulmonary circulation was soon to begin.
Great efforts have been made to identify noninvasive imaging methods that reflect the anatomical and functional status of the pulmonary circulation in an accurate and reproducible way. However, noninvasive assessment of the pulmonary circulation is quite difficult. First, because no method has sufficient sensitivity to assess the most important aspect of the pulmonary circulation and the seat of most diseases: the microcirculation. Second, because much of the information about the pulmonary vasculature is indirect. When diseased, the small blood vessels lose the mutual exclusivity between high compliance and low resistance.(3) The loss of elasticity and increased vascular impedance directly affect the RV.
Therefore, imaging of the RV might indirectly characterize the status of the pulmonary circulation. However, it is also difficult to study the RV, because of its anatomical position in relation to the left ventricle (LV), its complex crescent-shaped geometry, and its thin walls. All of this makes the assessment of systolic function, as well as the assessment of myocardial volumes and mass, a challenge for noninvasive imaging methods. The available methods employ different imaging technologies to generate information about pulmonary circulation. Chest X-ray is the simplest example. Despite its limitations, chest X-ray can reveal abnormalities that are not identified clinically. Echocardiography and CT of the pulmonary arteries (CT angiography), both of which are well-known and widely used, provide valuable information about the right heart and pulmonary arteries. Novel CT and magnetic resonance imaging (MRI) techniques have been found to play an important role in the dynamic, real-time assessment of the microcirculation. The innovative technique known as electrical impedance tomography (EIT) is a promising tool.
This article reviews the characteristics, limitations, and roles of the various imaging technologies currently available for the assessment of the pulmonary circulation, as well as for the diagnosis and management of PH and pulmonary thromboembolism (PTE).
Chest X-rayChest X-ray should be among the initial tests in the assessment of PH because it is readily available, is inexpensive, and facilitates the diagnosis.
Chest X-ray changes are seen in more than 85% of patients with a confirmed diagnosis of PH.(4) The major changes found include increased pulmonary artery diameter at the hilar level, in 78% of cases (Figure 1); peripheral tapering of vessels, in 62%; and hyperlucent lung periphery, in 9% (Figure 2). The pulmonary artery diameter at the hilar level can be determined by measuring the interlobar artery diameter. The maximum transverse diameter of the right interlobar artery, as measured from its lateral aspect to the air column of the intermediate bronchus, is 16 mm in men and 15 mm in women.(5) Because of the difficulty in assessing the left pulmonary artery by posteroanterior chest X-ray, the vessel should be assessed by lateral X-ray, beginning at the circular transparency created by the left upper lobe bronchus (which is visualized as being telescoped) and extending to the posterior margin of the vessel saddling the bronchus (Figure 1). The maximum limit of normality is 18 mm. A hilar-thoracic index (sum of the diameters of the pulmonary hila divided by that of the chest) above 38% is classically described, being present in approximately 75% of patients with PH (Figure 1).(6) Vascular calcification, usually located in the pulmonary artery trunk (PAT) or in its hilar branches, is rarely detected. This change is most commonly associated with congenital heart diseases and chronic central embolism.
Chest X-ray can facilitate the differential diagnosis with pulmonary parenchymal disease, heart failure, COPD, and kyphoscoliosis, as well as raising the suspicion of pulmonary thromboembolic disease when there are findings of oligemia, multiple areas of consolidation suggestive of pulmonary infarction, or asymmetry among the major pulmonary arteries (Figure 2).(7)
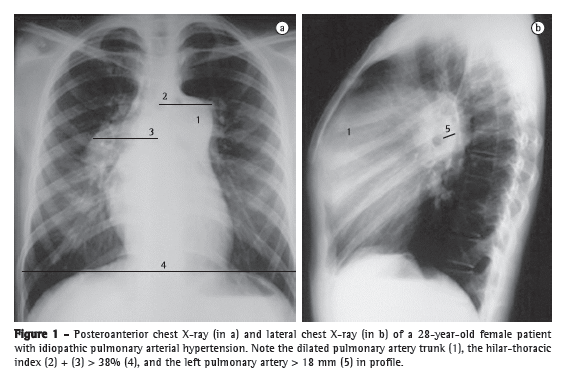
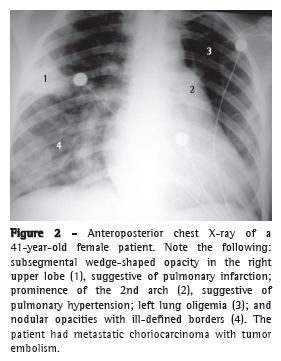
Although the presence of PH can be suggested by conventional X-ray, the specificity of this method and its degree of accuracy in estimating the severity of PH are controversial.(7) Several factors, such as the size of the patient, the distance between the X-ray tube and the film, and the distance between the pulmonary artery and the film, affect the interlobar artery measurements. Many of the parameters used are subjective, and the measurements correlate poorly with the degree of PH.(7) Nevertheless, routine chest X-ray plays a central role in the initial investigation of dyspnea, as well as in the initial evaluation of patients with suspected PH.
EchocardiographyTransthoracic Doppler echocardiography is the most sensitive noninvasive method for estimating pulmonary artery pressure (PAP) when there is suspicion of PH.(8)
However, RV ejection fraction and volumes cannot be calculated with the use of the mathematical equations usually employed to study the LV. Therefore, several echocardiographic parameters have been developed to assess RV function and the hemodynamics of the pulmonary artery. The most widely used of those methods is based on the identification of tricuspid regurgitation (TR). Measurement of TR provides an estimate of the pressure gradient in the right heart. The first studies involving continuous wave Doppler echocardiography were published in the mid-1980s.(9,10) The technique consists of measuring peak velocity of tricuspid regurgitation (VTR), which provides an estimate of the regurgitant flow from the RV to the right atrium (RA), as shown in Figure 3. With the use of the simplified Bernoulli equation (∆P = 4 × VTR2), it is possible to convert the flow measurement into an estimate of pressure. By adding this pressure gradient and an estimate of RA pressure, we obtain RV peak systolic pressure (RVSP), an approximation of the pulmonary artery systolic pressure (PASP), provided that there is no obstruction of the RV outflow tract. An RVSP > 35-40 mmHg is suggestive of PH.(10) In the presence of low regurgitant flow, the estimation of RVSP becomes less sensitive, because of the low signal intensity, which might lead to the underestimation of pressure values. However, the presence of extremely severe TR might also lead to the underestimation of pressure measurements, because equalizes the RA and RV pressures, resulting in a weak Doppler signal. Conversely, the method might also overestimate RVSP values.
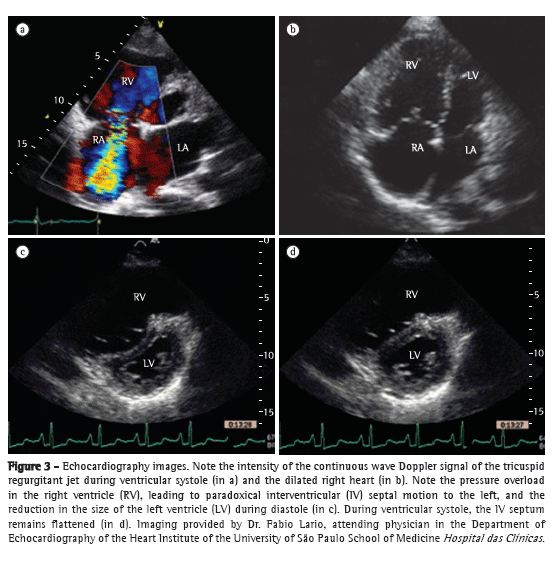
Another way to estimate PASP by echocardiography is by calculating the inferior vena cava compressibility index, as described by Pepi et al.(11) The authors found a strong correlation between RA pressure and the aforementioned index. With the use of a more reliable RA measurement, the percentage error in estimating PASP by the Bernoulli equation decreases, thus increasing the accuracy of the method.
In the 1990s, in order to improve the accuracy of the echo signal, the addition of intravenous contrast material to continuous wave Doppler echocardiography provided the opportunity to improve the sensitivity of the method. Materials such as 5% glucose microbubbles, sonicated human albumin, and indocyanine green increase echo intensity without affecting VTR. One group of authors(12) observed that, in a small patient sample (comprising 39 patients, most of whom had left heart disease), the estimation of PASP with the use of contrast resulted lower than the estimation of PASP with the use of right heart catheterization, the standard error for the latter being ± 5 mmHg in 51% of the patients and ± 10 mmHg in 82%.
At the beginning of the 21st century, two other indexes gained popularity in the echocardiographic evaluation of patients with PH. In a population of 26 patients with PH (known as primary PH at the time), Tei et al.(13)
described an index of combined systolic and diastolic function, known as the Tei index, which is obtained by dividing the sum of systolic and diastolic isovolumetric intervals by the ejection time. The Tei index was found to be a good predictor of survival in this population. Described in 1984 for the LV, the tricuspid annular plane systolic excursion (TAPSE) index reflects the longitudinal tricuspid annular motion during ventricular systole and correlates strongly with RV ejection fraction. Because TAPSE is a direct method that does not depend on ventricular geometry, it is quite reproducible and easy to obtain. In 2006, one group of authors(14) showed that a TAPSE index < 1.8 cm is indicative of more advanced RV dysfunction and correlates with a lower 19-month survival rate, especially in patients with pulmonary arterial hypertension (PAH).
Other echocardiographic parameters can also be used in the evaluation of PH. Measurement of TR at the opening of the pulmonary valve is used for estimating pulmonary artery diastolic pressure (PADP).(15) It is also possible to estimate pulmonary vascular resistance, which correlates with invasive hemodynamic measurement.(16) Atrial contraction and the subsequent increase in intracavitary pressure result in the formation of a characteristic wave, known as the A-wave. A less pronounced or absent A-wave on the PAP curve, probably attributable to increased PADP, is highly suggestive of PH. However, in patients with RV dysfunction, a normal A-wave does not rule out PH. Measurement the acceleration time of the pulmonary artery flow also has good accuracy in detecting increased PAP, representing an estimate of the mean pulmonary artery pressure (mPAP).(17) In addition, the finding of an increased RV systolic time interval is a highly specific echocardiographic sign of PH, albeit one with low sensitivity.(16) Other echocardiographic signs, such as right heart enlargement, pericardial effusion, RV dysfunction, paradoxical interventricular septal motion, and reduced LV filling volumes, also corroborate the presence of PH (Figure 3).(18) Provided that their limitations are taken into account and that they are evaluated and interpreted in the light of the clinical context, all these parameters can be useful in the diagnosis and follow-up of patients with PH.
Variations in PASP values can depending on the conditions and the characteristics of the population evaluated (age, level of exercise, level of fitness, and stress level). It has been reported that RVSP values are above 40 mmHg in 6% of subjects over 50 years of age and in 5% of those with a body mass index of 30 kg/m2.(18) Therefore, slightly increased values should be interpreted with caution.
In the context of acute PTE, echocardiography is highly valuable. Despite having no diagnostic power, except in cases of saddle PTE that can be visualized by Doppler, the method can predict mortality from thromboembolic events and possibly assist in the decision-making process regarding treatment. By providing morphological and functional information about the RV, echocardiography directly assesses the magnitude of the effect that pulmonary circulation obstruction has on the RV. Analyzing a cohort of a large population-based study,(19) one group of authors(20) observed that the finding of RV dysfunction on baseline echocardiography has a high positive predictive value for lower 30-day survival. The risk of death, reported in that study, was nearly twice as high in the patients with such dysfunction. In one systematic review,(21) the combined results of five studies evaluating the prognostic role of RV dysfunction as assessed by echocardiography revealed that the relative risk of death in patients with such dysfunction was 2.5 (95% CI: 1.2-5.5). This finding is of great importance because it identifies a subgroup of patients who are at high risk for complications and who, at admission, are stable from a hemodynamic standpoint.
In summary, echocardiography is widely available, inexpensive, and quite safe. Despite its limitations, echocardiography represents an important diagnostic and monitoring tool in PH and in PTE when used in combination with other markers.
Ventilation/perfusion scintigraphyThe major role of ventilation/perfusion scintigraphy in patients with PH is to distinguish chronic thromboembolic PH from other causes of PH.
The role of scintigraphy in the assessment of patients with PH, as well as in the assessment of patients with acute pulmonary embolism,(22) is well-established. A study conducted in 1994 involved 75 patients with documented causes of PH.(23) Of the 25 patients with chronic thromboembolic PH, 24 (96%) had high-probability scans and 1 (4%) had an intermediate-probability scan. Conversely, of the 35 patients with primary PH, 33 (94%) had low-probability scans, 1 (3%) had an intermediate-probability scan, and 1 (3%) had a high-probability scan. Of the 15 patients with non-thromboembolic secondary PH, the result was less accurate: 10 (67%) had low-probability scans, 3 (20%) had intermediate-probability scans, and 2 (13%) had high-probability scans. In view of the poorer yield in this third group of patients, the great importance of scintigraphy, in the context of PH, lies in the fact that it can rule out thromboembolic disease: a low-probability scan definitely excludes this disease. Another study showed that, in comparison with multidetector CT (MDCT), scintigraphy has far greater sensitivity for detecting chronic pulmonary embolism (96% vs. 51%).(24)
The typical pattern of scintigraphy findings in primary PH is that of poor perfusion (mottled pattern) in the periphery of the lung.(25) There is some correlation between the degree of perfusion heterogeneity in primary PH and patient prognosis.(25) Occasionally, multiple, small perfusion defects can be found in pulmonary veno-occlusive disease, pulmonary capillary hemangiomatosis, fibrosing mediastinitis, pulmonary vasculitis, and pulmonary artery sarcoma.
The most recent PH guidelines(26) recommend scintigraphy as the diagnostic method of choice for ruling out chronic PTE. In a patient with PH, a negative or low-probability scan virtually excludes chronic PTE, whereas a high-probability scan has a specificity of 96% for the diagnosis of chronic PTE in the presence of one or more segmental perfusion defects (Figure 4).(26)
CTObtaining images with the CT technique essentially consists of rotating a system comprising an X-ray tube and detectors around the body, and this introduces an innovation compared with routine X-ray: cross-sectional visualization of the various anatomical structures without overlap. However, because it is a system with weighty components, image acquisition speed has always been a major limitation of the method, presenting a particular hindrance in the assessment of the thoracic structures, which are constantly in motion.
The development of MDCT scanners, also known as multislice CT scanners, has revolutionized the history of CT, overcoming the temporal limitations of image acquisition. When compared with the conventional technology, MDCT allows a larger volume of tissues or structures to be represented in thinner slices (0.60-1.25 mm vs. 2-5 mm) at shorter intervals (2-8 s vs. 18-30 s), improving spatial resolution and reducing the occurrence of motion artifacts, all of this in a single breath hold.
In the assessment of the pulmonary circulation, the combination of the MDCT technology and automated injection of intravenous contrast material allows anatomical distinction of vascular structures and high-quality pulmonary vessel opacification. In addition, the nearly isotropic characteristic of voxels substantially improves image reformatting, optimizing multiplanar image acquisition.
In PH, CT angiography has diagnostic importance and is of great value in the etiological investigation.(27) The method allows the imaging assessment of the RV, PAT, and peripheral pulmonary circulation.
In CT angiography, the RV can be visualized with high spatial resolution. Interventricular septal dilatation, hypertrophy, flattening, and bowing are findings that strongly correlate with the presence of PH (Figure 5). Traditionally, however, the tomographic image of the RV is only static, causing a major limitation in its functional assessment. The use of electrocardiographic gating, that is, coupling of image acquisition with cardiac electrical phenomena, provides a partial solution to this limitation, allowing image reconstruction in any phase of the cardiac cycle as well as access to measurements of ventricular function and volumes.(28)
When technically well performed, CT angiography provides anatomically precise images of the PAT and allows its morphological assessment. Measurement of the PAT, with the use of axial slices, is performed at its bifurcation orthogonally to its longest axis and laterally to the ascending aorta. In a study of 32 cases of PH,(29) the 28.6 mm PAT diameter was strongly associated with the presence of PH as determined by invasive hemodynamic assessment. In a small population of 5 cases of HIV-related PH,(30) a PAT diameter > 29 mm showed a sensitivity and specificity, for the diagnosis of PH, of 87% and 89%, respectively; this aspect, combined with the presence of an artery-to-bronchus ratio > 1 in more than three lung lobes, was found to have a specificity of 100%.(31) Very similar findings were observed in a retrospective study of PH in patients with parenchymal lung disease.(31)
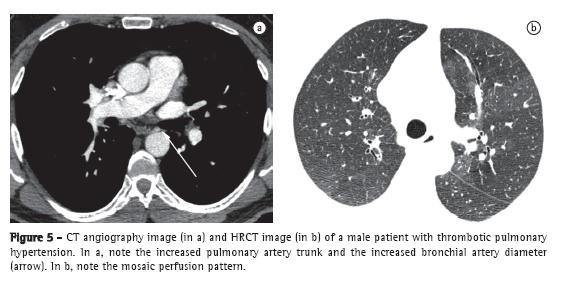
In the assessment of peripheral pulmonary circulation, CT angiography can uniformly access vessels of up to 2-3 mm with nearly constant opacification, high resolution, and no image overlap. This characteristic greatly contributes to the anatomical assessment of the pulmonary circulation. However, when it comes to visualization and quantification of the pulmonary microcirculatory flow, the method once again has functional limitations. The limited microcirculatory blood flow during vasoconstriction and vascular remodeling, characteristic of PH, can be visualized on HRCT scans (parenchymal window) as a mosaic perfusion pattern, which is present in patients with PH of various causes (Figure 5).(32) In some cases, there are also areas of increased lung parenchymal attenuation that are attributable to microcirculatory flow redistribution when the number and diameter of the vessels are increased in these regions. Both phenomena, mosaic perfusion and flow redistribution, are findings that are particularly more prevalent in chronic PTE. Dual-source CT, a new imaging technique in which images are obtained with two X-ray tubes, each with a different voltage, can access regional lung perfusion with greater accuracy, providing functional information.(33) This technology makes it possible to perform a CT angiography study and a perfusion study in a single test.
Having been widely studied, CT angiography has gained significant ground in the evaluation of acute PTE, becoming as accurate as pulmonary angiography but without its invasive nature. Some authors consider CT angiography to be the diagnostic imaging method of choice in the assessment of acute PTE.(34-36) This is explained by the various advantages of CT angiography: its high resolution allows the visualization of arteries and filling defects up to the distal portion of the pulmonary vasculature, reaching subsegmental vessels of 2-3 mm (Figure 6); it has excellent interobserver agreement, far superior to that of scintigraphy; its sensitivity is so high that a negative test result allows the safe discontinuation of anticoagulation therapy.(37) One must be alert, however, to the dangers inherent in the method. Technical or pathophysiological factors might lead to the interpretation of pseudo-filling defects, providing false-positive results. The possibility of motion artifacts in patients with tachypnea should be taken into consideration, as should the time interval between contrast injection and image acquisition, as well as the occurrence of hypoxic pulmonary vasoconstriction in poorly aerated regions. The advent of 64-channel scanners created another danger: the possibility of an excessive number of false-positive results. However, that possibility was ruled out in a recent study.(38)
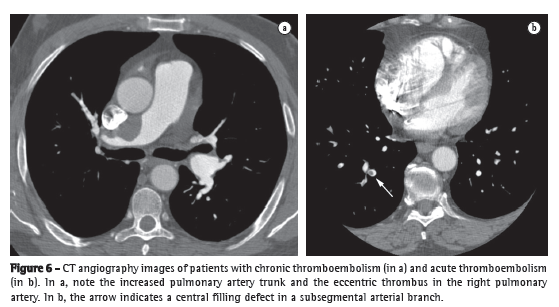
Because CT angiography analyzes the RV with high image resolution, it can serve as a marker of risk in patients with acute PTE, who have traditionally been stratified by echocardiography findings.(39) It is well established that the finding of RV dysfunction by echocardiography identifies a subgroup of patients at high risk for complications, worse prognosis, and higher mortality. A multiplanar reconstruction of a four-chamber view of the heart(40) or an axial view of the ventricles(41) allows the calculation of a simple ratio-RV diastolic diameter ÷ LV diastolic diameter = the RV/LV ratio. An RV/LV ratio > 0.90 correlates strongly with RV dysfunction as determined by echocardiography,(42) as well as showing potential cost-effectiveness and being a potential prognostic marker for CT angiography. In a recent systematic review,(21) the analysis of the combined results of two studies evaluating the prognostic role of RV dysfunction as assessed by tomography revealed that the relative risk of this dysfunction for predicting death was 2.3 (95% CI: 0.90-5.98). In cases of distal chronic PTE, however, CT angiography has limitations, and ventilation/perfusion scintigraphy has become the test of choice.(43)
MRIThe MRI technique holds great prospects for the assessment of the pulmonary circulation. The method comprises excellent characteristics, such as low operator dependence, high accuracy, and good reproducibility.(44)
The MRI technique can be used for the assessment of the lung parenchyma and pulmonary circulation, as well as for the dynamic analysis of the heart. In the assessment of the lung parenchyma, MRI faces some challenges: low proton density of the lung parenchyma, resulting in a low signal/noise ratio; signal loss during physiological motion of the intrathoracic organs; and the combination of air and soft tissues, which increases its susceptibility to artifacts. Despite these problems, some adjustments in the form of image acquisition make MRI an interesting tool in the assessment of the lung parenchyma. Some authors have suggested that, for specific applications, MRI findings are equivalent to CT findings, although MRI should not yet be used for the aforementioned purpose in routine clinical practice.(45) There is as yet no systematic evaluation of MRI as a means of assessing the lung parenchyma of patients with PH.
Since 1993, when the technique known as enhanced three-dimensional magnetic resonance arteriography (MRA) was published,(46) there have been many advances in the assessment of the intrathoracic vessels. This technique consists of the injection of paramagnetic contrast material and ultra-fast T1-weighted image acquisition, resulting in high spatial resolution during periods of apnea lasting less than 30 s.
Chief among the useful functions of pulmonary MRA is its great importance in detecting acute or chronic pulmonary embolism. In this context, a study published in 2002(47) evaluated 141 patients with suspected PTE and with abnormal perfusion scintigraphy findings. The sensitivity of MRA for segmental and central embolism (84% and 100%, respectively) was found to be comparable to that of pulmonary arteriography. However, for subsegmental embolism, the sensitivity of MRA was low (40%). Other studies(48,49) have confirmed these findings, and MRA plays a very interesting role in patients with suspected PTE who have contraindications to the use of iodinated contrast material or who want to avoid ionizing radiation. Another promising use of MRA is in the noninvasive evaluation of patients with PAH. One group of authors(50) investigated the use of MRI in the evaluation of responders to vasodilator testing with inhaled NO. When analyzing the mean pulmonary artery distensibility, those authors found a sensitivity of 100% in the identification of responders, with a specificity of 56% when compared with
hemodynamic evaluation with a pulmonary artery catheter.
The functional assessment of microcirculation is also possible with the use of MRI. One group of authors(51) compared perfusion changes detected on MRI with those detected on pulmonary perfusion scintigraphy in patients with pulmonary embolism, pneumonia, and COPD. The tests were evaluated by two observers, and there was moderate concordance (kappa coefficient range: 0.52-0.57) between the two techniques.
The most common use of MRI in PH remains the assessment of the RV. This technique allows the acquisition of high-resolution dynamic images of myocardial contraction and does not depend on anatomical conditions (pulmonary emphysema, obesity, etc.) as does echocardiography.
In patients with PH, cardiac MRI is especially important for the assessment of the following parameters (Figure 7):
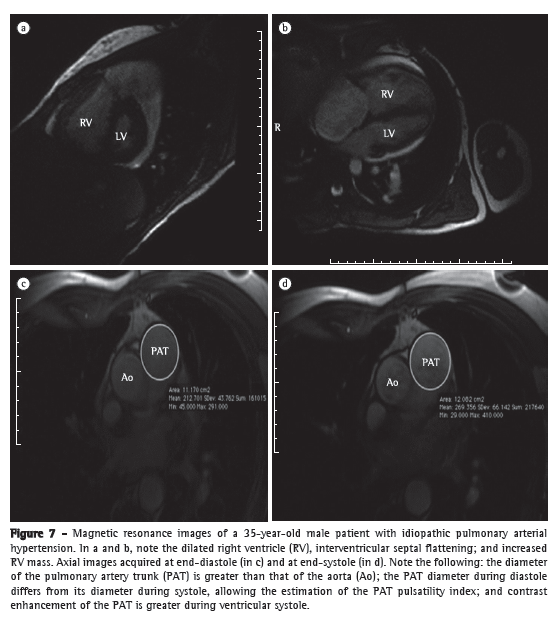
Ventricular morphology-CT images that make it possible to determine the size and volume of the heart chambers, as well as wall thickness, and muscle mass. End-diastolic RV volume is significantly increased in PH. One group of authors(52) studied the role of cardiac MRI in the prognostic evaluation of patients with PH and observed that patients with increased diastolic RV volume and reduced systolic RV volume or reduced diastolic LV volume have a worse prognosis at 12-month follow-up. The study suggests that MIR can also be used as a tool for monitoring and evaluating treatment response.(52) In addition, MRI can analyze RV mass, which, during increased afterload, is significantly increased. Evidence suggests that RV mass index correlates well with mPAP.(53)
Ventricular function-dynamic images that reproduce the entire cardiac cycle can be obtained with electrocardiographic gating and have high accuracy and reproducibility in the analysis of ventricular function.(54) Another interesting tool is the evaluation of diastolic RV dysfunction and the behavior of the RV following treatment.
Interventricular septal configuration-distortions in the normal morphology of the interventricular septum can be observed in patients with PH. These quantifiable changes correlate with mPAP and prognosis.(55)
Flow analysis-volumetric flow measurements can be obtained with the use of contrast material and sequential image acquisition, which provides data for the determination of cardiac output, the evaluation of valvular regurgitation, the assessment of diastolic ventricular filling, and the quantification of cardiac shunts. Cardiac MRI has advantages over thermodilution in the acquisition of cardiac output measurements because it is noninvasive, it is less dependent on changes from one cardiac cycle to another, and it is not as strongly affected by TR.(56)
Myocardial viability assessment-the assessment of myocardial contrast after gadolinium infusion can directly show nonviable areas of the myocardium. One group of authors(57) described a pattern of myocardial contrast enhancement in patients with PH. Delayed contrast enhancement was present within the RV insertion points and interventricular septum of 23 of the 25 patients studied. The extent of delayed contrast enhancement correlated with worse RV function.
In addition, the method shows potential for the molecular assessment of the pulmonary circulation and the heart. It is possible, for instance, to evaluate and quantify areas of apoptosis and the degree of apoptosis in vivo.(58)
In Brazil, MRI is quite costly and is not widely available. Although preliminary in nature, the findings related to the use of MRI as a diagnostic and prognostic tool in the assessment of PH seem promising.
EITThe concept of electrical impedance can be defined in various ways: the relationship between the voltage gradient generated in an electrical circuit and the resulting electrical current; the combination of resistance and reactance; and the total opposition that a circuit offers to the flow of alternating current at a particular frequency. Essentially, higher resistance (or lower conductance) in a circuit, structure, or tissue translates to higher electrical impedance values.
A system developed in the 1980s in the United Kingdom makes it possible to measure the impedance of a structure or tissue by placing pairs of electrodes in rows on its surface. Sending electrical currents of low amperage and low frequency through these electrodes generates a voltage gradient, which, in turn, results in an electron flow that can be estimated or measured. A mathematical algorithm for image reconstruction can transform the measured value of electrical impedance into pixels, the basic unit of the composition of an image. With the cross-sectional distribution of the electrodes in rows, it is possible to obtain a cross-sectional image of the distribution of electrical impedance within the geometric plane defined by the electrodes. This system is known as EIT.
The biological properties of the chest provide a valuable opportunity for assessment with the use of EIT, because air and blood, two materials with opposite resistances, share the same compartment and vary their volumes rhythmically during the cardiorespiratory cycle. The periodic change in intrathoracic blood volume during cardiac work and the distension of pulmonary microcirculation resulting from the blood flow through the pulmonary vessels are associated with the cyclic change in the electrical properties of the lung parenchyma over time. The increased blood volume in the lung parenchyma results in a 1% decrease in lung electrical impedance (blood being five time less resistant than is air).
Regional changes in blood flow can be visualized by EIT in two ways. The first, which is based on the indicator dilution technique, involves the use of an electrically conducting fluid as contrast material, similarly to CT and MRI studies. The second approach analyzes the changes in the lung parenchyma impedance resulting from the change in systolic volume during the cardiac cycle, cycle by cycle. Coupling of image acquisition with the R-wave on electrocardiogram (the ECG-gated technique) filters ventilation-related fluctuations, obtaining only those that are due to circulation. The resulting image is likely to reflect pulmonary circulation pulsatility or distensibility.
An excellent model of disease in the pulmonary microcirculation is PAH, in which vascular remodeling directly affects the distensibility properties of microcirculation. When vascular compliance is reduced, with consequent accommodation of lower volumes, the electrical impedance change in PH is likely to be decreased as well. In one study,(59) EIT was used in patients with PH for determining vascular response to vasodilator testing with epoprostenol during invasive hemodynamic assessment. Of the 8 patients evaluated, 7 did not meet the vasodilator response criteria, EIT showing no impedance change in those 7. In the lone responder, the increase in impedance change was found to correlate strongly with decreases in mPAP and pulmonary vascular resistance, regardless of the increase in systolic volume. In another study,(60) the differences in the impedance of the pulmonary circulation were analyzed in 21 patients with idiopathic PAH (IPAH) and 30 healthy subjects. The authors observed a reduction in impedance change in the IPAH group, which provides support for the use of EIT in the assessment of the pulmonary circulation and of PH.
In Brazil, a 32-electrode EIT scanner is being developed for the assessment of lung perfusion. In the context of IPAH, the images obtained with this new scanner (Figure 8) are encouraging, demonstrating not only a reduction in electrical impedance, as in the two aforementioned studies, but also a change in the impedance wave morphology, which might correlate with the pulse wave of the pulmonary circulation.
References1. Cournard A, Riley RL, Breed ES et al. Measurement of cardiac output in man using the technique of catheterization of right auricle or ventricle. J Clin Invest. 1945; 24 (1): 106-16.
2. Steckelberg JM, Vliestra RE, Ludwig J, Mann RJ. Werner Forssmann (1904--1979) and his unusual success story. Mayo Clin Proc. 1979; 54 (11): 746-8.
3. Souza R. Assessment of compliance in pulmonary arterial hypertension. Eur Heart J. 2008; 29: 1603-4.
4. Paciocco G, et al. Pulmonary hypertension. Chest. 1998; 114 (4S): 375S-6S.
5. Lupi HE, et al. Indications and radiological measurements in the evaluation of pulmonary artery hypertension. Arch Inst Cardiol Mex. 1975; 45: 34-42.
6. Lupi E, et al. A radiologic index of pulmonary arterial hypertension. Chest. 1975; 68 (1): 28-31.
7. Terence KT, John RM. Diagnosis of pulmonary arterial hypertension. Clin Chest Med. 2007; 28 (1): 59-73.
8. Celermajer DS, Marwick T. Echocardiographic and right heart catheterization techniques in patients with pulmonary arterial hypertension. Int J Cardiol. 2008; 125: 294-303.
9. Berger M, Haimowitz A, Van Tosh A et al. Quantitative assessment of pulmonary hypertension in patients with tricuspid regurgitation using continuous wave Doppler ultrasound. J Am Coll Cardiol. 1985; 6: 359.
10. Kitabatake, Inoue M, Asao M, et al. Noninvasive evaluation of pulmonary hypertension by a pulsed Doppler technique. Circulation. 1983;
68: 302-9
11. Pepi M, Tamborini G, Sganzerla P. The noninvasive estimation of right atrial pressure improves the Doppler evaluation of the pulmonary systolic pressure. Cardiologia. 1991; 36: 117-22.
12. Tokushima T, Utsunomiya T, Yoshida K et al. Estimation of the systolic pulmonary arterial pressure using contrast-enhanced continuous-wave Doppler in patients with trivial tricuspid regurgitation. Jpn Heart J. 1999; 40: 311-20.
13. Tei C, Dujardin KS, Hodge DO, et al. Doppler Echocardiographic index for assessment of global right ventricular function. J Am Soc Echocardiogr. 1996; 9: 838-47.
14. Forfia Pr, Fisher MR, Mathai FC, et al. Tricuspid annular displacement predicts survival in pulmonary hypertension. Am J Respir Crit Care Med. 2006; 174: 1034-41.
15. Lanzarini L, Fontana A, Lucca E, et al. Noninvasive estimation of both systolic and diastolic pulmonary artery pressure from Doppler analysis of tricuspid regurgitant velocity spectrum in patients with chronic heart failure. Am Heart J. 2002; 144: 1087-94.
16. Selimovic N, Rundqvist B, Bergh C-H, et al. Assessment of pulmonary vascular resistance by Doppler echocardiography in patients with pulmonary arterial hypertension. J Heart Lung Transplant. 2007; 26: 927-34.
17. Bossone E, Duong-Wagner TH, Paciocco G, et al. Echocardiographic features of primary pulmonary hypertension. J Am Soc Echocardiogr. 1999; 12: 655-62.
18. McQuillan BM, Picard MH, Leavitt M, Weyman AE. Clinical correlates and reference intervals for pulmonary artery systolic pressure among echocardiographically normal subjects. Circulation. 2001; 104: 2797-802.
19. Goldhaber SZ, Visani L, De Rosa M. Acute pulmonary embolism: clinical outcomes in the International Cooperative Pulmonary Embolism Registry (ICOPER). Lancet. 1999; 353: 1386-9.
20. Kucher N, Rossi E, De Rosa M, Goldhaber SZ. Prognostic Role of Echocardiography Among Patients With Acute Pulmonary Embolism and a Systolic Arterial Pressure of 90 mm Hg or Higher. Arch Intern Med. 2005; 165: 1777-81.
21. Sanchez O, Trinquart L, Colombet I, et al. Prognostic value of right ventricular dysfunction in patients with haemodynamically stable pulmonary embolism: a systematic review. Eur Heart J. 2008; 29: 1569-77.
22. Value of the ventilation/perfusion scan in acute pulmonary embolism. Results of the prospective investigation of pulmonary embolism diagnosis (PIOPED). The PIOPED Investigators. JAMA. 1990; 263(20): 2753-9.
23. Worsley DF, Palevsky HI, Alavi A. Ventilation-Perfusion Lung Scanning in the Evaluation of Pulmonary Hypertension. J Nucl Med. 1994; 35(5): 793-6.
24. Tunariu N et al. Ventilation-Perfusion Scintigraphy Is More Sensitive than Multidetector CTPA in Detecting Chronic Thromboembolic Pulmonary Disease as a Treatable Cause of Pulmonary Hypertension. J Nucl Med. 2007; 48(5): 680-4.
25. Fukuchi K, et al. Quantitative Analysis of Lung Perfusion in Patients with Primary Pulmonary Hypertension. J Nucl Med. 2002; 43(6): 757-61.
26. Hoeper MM, Barberà JA, Channick RN. Diagnosis, assessment, an treatment of non-pulmonary arterial hypertension
pulmonary hypertension. J Am Coll Cardiol. 2009; 54: S85-96.
27. Ley S, Kreitner K-F, Fink C, et al. Assessment of pulmonary
hypertension by CT and MR imaging. Eur Radiol. 2004; 14: 359-68.
28. McLure LER, Peacock AJ. Imaging of the heart in pulmonary hypertension. Int J Clin Pract. 2007; 65: 15S-26S.
29. Kuriyama K, Gamsu G, Stern RG, et al. CT-determined pulmonary artery diameters in predicting pulmonary hypertension. Invest Radiol. 1984; 19: 16-22.
30. Bugnone AN, Viamonte M Jr, Garcia H. Imaging findings in human immunodeficiency virus-related pulmonary hypertension: report of five cases and review of the literature. Radiology. 2002; 223: 820-827.
31. Tan RT, Kuzo R, Goodman LR, et al. Utility of CT scan evaluation for predicting pulmonary hypertension in patients with parenchymal lung disease. Chest. 1998; 113: 1250-6.
32. Sheerick AD, Swensen SJ, Hartman TE. Mosaic pattern of lung attenuation on CT scans: frequency among patients with pulmonary artery hypertension of different causes. ARJ. 1997; 169: 79-82.
33. Thieme SF, Becker CR, Hacker M, Nikolaou K, Reiser MF, Johnson TR. Dual energy CT for the assessment of lung perfusion--correlation to scintigraphy. Eur J Radiol. 2008; 68(3): 369-74.
34. Remy-Jardin M, Ghaye B, Remy J. Spiral computed tomography angiography of pulmonary embolism. Eur Resp Mon. 2003; 27: 124-43.
35. Ferreti GR, Bosson JL, Buffaz PD, et al. Acute pulmonary embolism: role of helical CT in 164 patients with intermediate probability in ventilation-perfusion scintigraphy and normal results at duplex US of the legs. Radiology. 1997; 205: 453-8.
36. Mayo JR, Remy-Jardin M, Muller NL, et al. Pulmonary embolism: prospective comparison of spiral CT and ventilation-perfusion scintigraphy. Radiology. 1997; 205: 447-52.
37. Goodman LR, Lipchick RJ, Kuzo RS, et al. Subsequent pulmonary embolism: risk after a negative helical CT pulmonary angiogram - prospective comparison with scintigraphy. Radiology. 2000; 215: 535-42.
38. Sohnsa C, Amarteifioa E, Sossallab S, Heuserc M, Obenauera S. 64-Multidetector-row spiral CT in pulmonary embolism with emphasis on incidental findings. Clinical Imaging. 2008; 32: 335-41.
39. Quiroz R, Kucher N, Schoepf UJ, et al. Right ventricular enlargement on chest computed tomography: prognostic role in acute pulmonary embolism. Circulation. 2004; 109: 2401-4.
40. Kamel EM, Schmidt S, Doenz F, et al. Computed Tomographic Angiography in Acute Pulmonary Embolism: Do We Need Multiplanar Reconstructions to Evaluate the Right Ventricular Dysfunction? J Comput Assist Tomogr. 2008; 32: 438-43.
41. Schoepf UJ, Kucher N, Kipfmueller F, et al. Right ventricular enlargement on chest computed tomography: a predictor of early death in acute pulmonary embolism. Circulation. 2004; 110: 3276-8.
42. van der Meer RW, Pattynama PM, van Strijen MJ, et al. Right ventricular dysfunction and pulmonary obstruction index at helical CT: prediction of clinical outcome during 3-month follow-up in patients with acute pulmonary embolism. Radiology. 2005; 235: 798-803.
43. Dias BA, Jardim C, Hovnanian A, Fernandes CJ, Souza R. Chronic Thromboembolic Pulmonary Hypertension: Diagnostic Limitations. J Bras Pneumol. 2008; 34 (7): 532-6.
44. Ley S, et al. Assessment of pulmonary hypertension by CT and MR imaging. Eur Radiol. 2004; 14: 357-8.
45. Biederer J, et al. Lung Morphology: Fast MR Imaging Assessment with a Volumetric Interpolated Breath-Hold Technique: Initial Experience with Patients. Radiology. 2003; 226: 242-9.
46. Prince MR, et al. Dynamic gadolinium-enhanced three-dimensional abdominal MR arteriography. J Magn Reson Imaging. 1993; 3: 877-81.
47. Oudkerk M, et al. Comparison of contrast-enhanced magnetic resonance angiography and conventional pulmonary angiography for the diagnosis of pulmonary embolism: a prospective study. Lancet. 2002; 359: 1643-7.
48. Meaney JFM, et al. Diagnosis of Pulmonary Embolism with Magnetic Resonance Angiography. N Engl J Med. 1997. 336: 1422-7.
49. Ersoy H, et al. Time-Resolved MR Angiography: A Primary Screening Examination of Patients with Suspected Pulmonary Embolism and Contraindications to Administration of Iodinated Contrast Material. Am J Roentgenol. 2007; 188: 1246-54.
50. Jardim C, et al. Pulmonary artery distensibility in pulmonary arterial hypertension: an MRI pilot study. Eur Respir J. 2007; 29: 476-81.
51. Amundsen T, et al. Perfusion abnormalities in pulmonary embolism studied with perfusion MRI and ventilation-perfusion scintigraphy: An intra-modality and inter-modality agreement study. J Magn Reson Imaging. 2002 15: 386-94.
52. van Wolferen SA, et al. Prognostic value of right ventricular mass, volume, and function in idiopathic pulmonary arterial hypertension. Eur Heart J. 2007; 28: 1250-7.
53. Saba TS, et al. Ventricular mass index using magnetic resonance imaging accurately estimates pulmonary artery pressure. Eur Respir J. 2002; 20: 1519-24.
54. Hoeper MM, et al. Evaluation of Right Ventricular Performance With a Right Ventricular Ejection Fraction Thermodilution Catheter and MRI in Patients With Pulmonary Hypertension. Chest. 2001; 120: 502-7.
55. Roeleveld RJ, et al. Interventricular Septal Configuration at MR Imaging and Pulmonary Arterial Pressure in Pulmonary Hypertension. Radiology. 2005; 234: 710-7.
56. Mousseaux E, et al. Pulmonary Arterial Resistance: Noninvasive Measurement with Indexes of Pulmonary Flow Estimated at Velocity-encoded MR Imaging-Preliminary Experience. Radiology. 1999; 212: 896-902.
57. Blyth KG, et al. Contrast enhanced-cardiovascular magnetic resonance imaging in patients with pulmonary hypertension. Eur Heart J. 2005 26: 1993-9.
58. Sosnovik DE, et al. Magnetic resonance imaging of cardiomyocyte apoptosis with a novel magneto-optical nanoparticle. Magn Reson Med. 2005; 54: 18-24.
59. Smit HJ, Vonk Noordegraaf A, Marcus JT, et al. Determinants of pulmonary perfusion measured by electrical impedance tomography. Eur J Appl Physiol. 2004; 92: 45-9.
60. Smit HJ, Vonk Noordegraaf A, Boonstra A, Vries PM, Postmus PE. Assessment of the pulmonary volume pulse in idiopathic pulmonary arterial hypertension by means of electrical impedance tomography. Respiration. 2006, 73: 597-602.
* Study carried out by the Pulmonary Hypertension Group, Department of Pulmonology, Instituto do Coração, Hospital das Clínicas da Faculdade de Medicina da Universidade de São Paulo - InCor/HCFMUSP, Heart Institute/University of São Paulo School of Medicine Hospital das Clínicas - São Paulo, Brazil.
Financial support: None.
Correspondence to: Rogério Souza. Grupo de Hipertensão Pulmonar, Disciplina de Pneumologia, Instituto do Coração, HCFMUSP, Avenida Dr. Enéas de Carvalho Aguiar, 44, 5º andar, Bloco II, CEP 05403-000, São Paulo, SP, Brasil.
Tel/Fax: 55 11 3069-5695. E-mail: rogerio.souza@incor.usp.br
Submitted: 2 May 2010. Accepted, after review: 11 May 2011.
About the authorsAndré Hovnanian
Physician. Pulmonary Hypertension Group, Department of Pulmonology, Instituto do Coração, Hospital das Clínicas da Faculdade de Medicina da Universidade de São Paulo - InCor/HCFMUSP, Heart Institute/University of São Paulo School of Medicine Hospital das Clínicas - São Paulo, Brazil.
Eduardo Menezes
Physician. Pulmonary Hypertension Group, Department of Pulmonology, Instituto do Coração, Hospital das Clínicas da Faculdade de Medicina da Universidade de São Paulo - InCor/HCFMUSP, Heart Institute/University of São Paulo School of Medicine Hospital das Clínicas - São Paulo, Brazil.
Susana Hoette
Physician. Pulmonary Hypertension Group, Department of Pulmonology, Instituto do Coração, Hospital das Clínicas da Faculdade de Medicina da Universidade de São Paulo - InCor/HCFMUSP, Heart Institute/University of São Paulo School of Medicine Hospital das Clínicas - São Paulo, Brazil.
Carlos Jardim
Attending Physician. Pulmonary Hypertension Group, Department of Pulmonology, Instituto do Coração, Hospital das Clínicas da Faculdade de Medicina da Universidade de São Paulo - InCor/HCFMUSP, Heart Institute/University of São Paulo School of Medicine Hospital das Clínicas - São Paulo, Brazil.
Dany Jasinowodolinski
Physician. Pulmonary Hypertension Group, Department of Pulmonology, Instituto do Coração, Hospital das Clínicas da Faculdade de Medicina da Universidade de São Paulo - InCor/HCFMUSP, Heart Institute/University of São Paulo School of Medicine Hospital das Clínicas - São Paulo, Brazil.
Rogério Souza
Tenured Professor. Department of Pulmonology, Instituto do Coração, Hospital das Clínicas da Faculdade de Medicina da Universidade de São Paulo - InCor/HCFMUSP, Heart Institute/University of São Paulo School of Medicine Hospital das Clínicas - São Paulo, Brazil.