ABSTRACT
Magnetic resonance imaging (MRI) of the lung has progressed tremendously in recent years. Because of improvements in speed and image quality, MRI is now ready for routine clinical use. The main advantage of MRI of the lung is its unique combination of structural and functional assessment in a single imaging session. We review the three major clinical indications for MRI of the lung: staging of lung tumors; evaluation of pulmonary vascular disease; and investigation of pulmonary abnormalities in patients who should not be exposed to radiation.
Keywords:
Magnetic resonance imaging; Lung; Lung diseases; Carcinoma, non-small-cell lung; Lung diseases, interstitial; Pneumonia.
RESUMO
A ressonância magnética (RM) de pulmão progrediu tremendamente nos últimos anos. Devido a melhorias na velocidade e na qualidade de imagens, a RM de pulmão hoje está pronta para a utilização clínica de rotina. A principal vantagem da RM de pulmão é a sua combinação exclusiva de avaliação estrutural e funcional em uma única sessão de imagens. Revisamos aqui as três principais indicações clínicas da RM de pulmão: o estadiamento de tumores pulmonares, a avaliação de doença vascular pulmonar e a investigação de anormalidades pulmonares em pacientes que não podem ser expostos à radiação.
Palavras-chave:
Imagem por ressonância magnética; Pulmão; Pneumopatias; Carcinoma pulmonar de células não pequenas; Doenças pulmonares intersticiais; Pneumonia. Imagem por ressonância magnética; Pulmão; Pneumopatias; Carcinoma pulmonar de células não pequenas; Doenças pu
IntroductionMagnetic resonance imaging (MRI) of the lung was first attempted in the 1980s.(1) In the following years, efforts were made to improve its performance and quality. However, there were a number of general difficulties that could not initially be overcome: low signal intensity due to low proton density; susceptibility artifacts caused by multiple air-tissue interfaces; and motion artifacts (respiratory, cardiac, and vascular). Subsequent technological developments improved the quality and broadened the application of the procedure.(2,3) It is now possible to perform magnetic resonance angiography (MRA) of the pulmonary arteries with high spatial resolution, for the diagnosis of suspected pulmonary embolism or pulmonary hypertension, as well as multiphase MRA with high temporal resolution, for the depiction of vascular territories and of pulmonary perfusion (4)
Most lung diseases are accompanied by an increase in the quantity of tissue, cells, or blood within the lungs, which facilitates MRI because it makes more protons available to generate a signal.(5-8) Therefore, structural MRI is feasible, and tissue can be characterized by the differences observed among T1-weighted, T2-weighted, and other types of sequences, as well as by the assessment of contrast-enhanced images. In the staging of lung cancer, MRI has proven to be superior to CT, and there is evidence that it is also superior in the characterization of pulmonary nodules larger than 1 cm in diameter.(6-8) In addition, MRI has advantages in the characterization of pulmonary infiltrates, especially in patients with neutropenic fever, although such use might not be cost-effective.(9) Currently, the most relevant clinical application of MRI of the lung is in the staging of lung cancer patients, for which purpose MRI is as accurate as is 18F-fluorodeoxyglucose positron emission tomography with computed tomography (FDG-PET/CT).(7)
The clinical indications for MRI of the lung are related to three major objectives: the staging of lung tumors; the assessment of pulmonary vascular disease; and the investigation of any pulmonary abnormalities in patients who should not be exposed to radiation. Here, we review those indications.
Clinical indications for MRI of the lungDetection and characterization of pulmonary nodulesIt is estimated that, in the United States, there are approximately 150,000 new cases of pulmonary nodules seen on routine chest X-rays every year.(9) One recent meta-analysis reported that dynamic CT and MRI, both of which are noninvasive methods, are equally accurate in distinguishing between malignant and benign solitary pulmonary nodules, the differences between the two tests being insignificant.(10) The authors of that meta-analysis found that, for the 10 dynamic CT studies, MRI had a pooled sensitivity of 93% (95% CI: 0.88-0.97) and a pooled specificity of 76% (95% CI: 0.68-0.97).(10) Koyama et al.(11) reported that non-contrast-enhanced MRI of the lung is as efficient as is thin-section multidetector CT in detecting malignant nodules. The authors also found that the overall nodule detection rate in each MRI sequence (82.5%) was significantly lower than was that of multidetector CT (97.0%), although there was no significant difference between the two techniques in terms of the detection rate for malignant nodules.(11) Figure 1 shows a comparison of images obtained with those two modalities.
Tumor-node-metastasis stagingIn the tumor-node-metastasis (TNM) staging system, the T stage (depth of tumor invasion) is the primary determinant of the gravity of neoplasia.(12) For the depiction of the pericardium, heart, and mediastinal vessels, MRI is superior to CT and can therefore be indicated in specific situations, such as superior vena cava obstruction, invasion of the myocardium, or the spread of the tumor into the left atrium via the pulmonary veins.(13) In addition, MRI can allow lung cancer to be distinguished from secondary changes due to atelectasis or pneumonitis.(12) In T2-weighted MRI sequences, post-obstructive atelectasis and pneumonitis often show higher signal intensity than does the central tumor (Figure 2).(12)
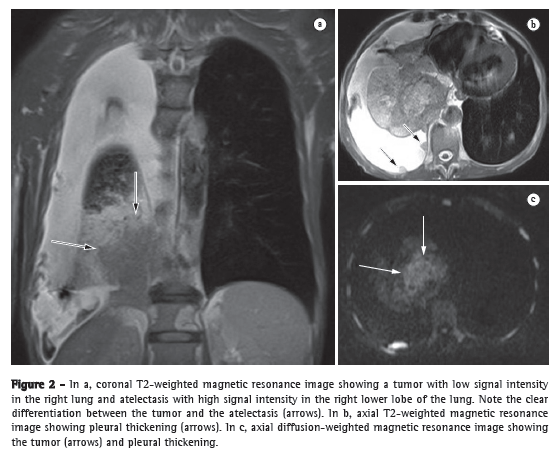
The accurate assessment of mediastinal lymph nodes is essential in selecting the appropriate treatment plan for patients with non-small cell lung cancer (NSCLC). Figure 3 shows CT and MRI scans of a suspicious lymph node. Ohno et al.(7) conducted a prospective study of 115 consecutive NSCLC patients undergoing CT, short-tau inversion-recovery turbo spin-echo (STIR-TSE) imaging, and FDG-PET/CT, as well as surgical and pathological examination. The authors found that, on a per-patient basis, the quantitative sensitivity and accuracy of STIR-TSE imaging (90.1% and 92.2%, respectively) were significantly higher than were the quantitative sensitivity, qualitative sensitivity, quantitative accuracy, and qualitative accuracy of co-registered FDG-PET/CT (76.7%, 74.4%, 83.5%, and 82.6%, respectively).
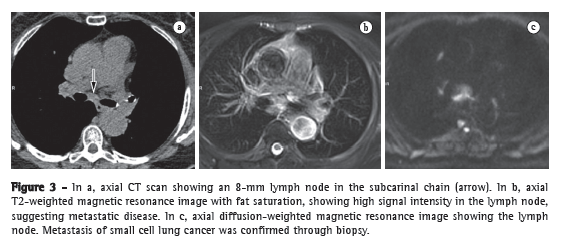
Because it provides higher efficacy in TNM stage determinations than do conventional staging methods, integrated PET/CT seems to be a first-line lung cancer staging tool. However, MRI has come to play an increasingly more important role in this setting. Figure 4 shows the ability of an MRI scan to clearly depict local metastases of a lung tumor. It has been reported that PET/CT fails to reveal microscopic metastases in approximately 20% of patients undergoing surgical therapy.(12) Previous studies have demonstrated that whole-body MRI provides acceptable accuracy, and that its efficacy in lung cancer staging is comparable to that of PET/CT.(13) Each of those two imaging modalities has been shown to have its advantages,(12) whole-body MRI being better for detecting brain and liver metastases, whereas PET/CT is better for detecting lymph node and soft-tissue metastases. For the evaluation of areas that have been submitted to radiation therapy, one promising technique is diffusion.(12) Whole-body MRI with diffusion-weighted imaging can be used for the assessment of the M (metastasis) stage in NSCLC patients and has been shown to be as accurate as is PET/CT.(14)
Pulmonary thromboembolic diseasePulmonary thromboembolic disease refers to the condition in which a blood clot or clots (thrombus or multiple thrombi) migrate from the systemic circulation to the pulmonary vasculature.(15) Although pulmonary embolism is the third leading acute cardiovascular disease (after myocardial infarction and stroke), it often goes undetected and is therefore responsible for thousands of deaths every year.(16)
Substantial technical advances in pulmonary MRA have been introduced in recent years (Figures 5 and 6). Continued improvements are ongoing and include the use of parallel imaging, view sharing, time-resolved echo-shared angiography,(17-19) and pulmonary perfusion. These techniques have shortened MRA acquisition time, made it less susceptible to motion artifacts, and improved spatial resolution. One study showed that a combined magnetic resonance protocol (progressing from real-time MRI to perfusion MRI to MRA) is reliable and sensitive, producing results similar to those obtained with 16-slice multidetector CT.(18)
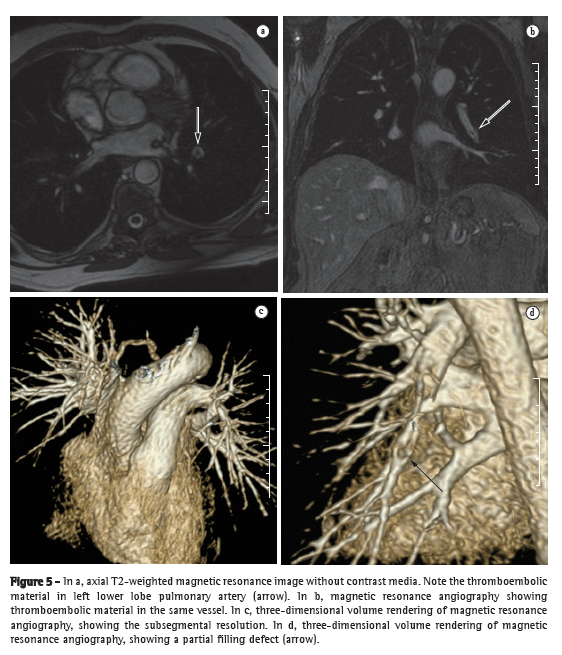
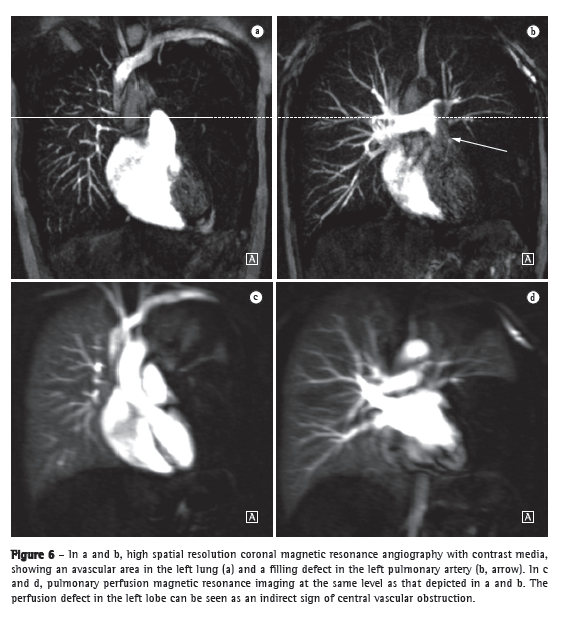
In 2003, Stein et al.(20) conducted a meta-analysis of the use of gadolinium-enhanced MRI for the depiction of acute pulmonary embolism. The authors used conventional pulmonary angiography as the reference standard. They found that the reported sensitivity of the procedure covered a broad range (77-100%) and that the reported specificity was uniformly high (95-98%).(20) In the most recent of the studies evaluated in that meta-analysis, Oudkerk et al.(21) showed that the sensitivity of contrast-enhanced MRI for pulmonary embolism was 100% in the central and lobar arteries; 84% in the segmental arteries; and only 40% in the subsegmental branches.
In cases of suspected acute pulmonary embolism, MRI with a state-of-the-art, three-component protocol (true fast imaging with steady-state precession; perfusion; and MRA with parallel acquisition) has recently been shown to be highly accurate.(18) The reported per-patient sensitivity and specificity, respectively, are 85% and 98% for the true fast imaging sequence; 100% and 91% for the perfusion sequence; and 77% and 100% for the MRA. The combined protocol has a reported sensitivity of 100% and a reported specificity of 93%. Although MRI and MRA are specific, they have low sensitivity, particularly for subsegmental pulmonary embolism. Overall, the combined MRI protocol has been found to be more reliable and sensitive than is 16-slice multidetector CT.(18) The average MRI examination time is reported to be approximately 10 min.(18)
Pulmonary hypertensionThe use of MRI allows the comprehensive assessment of pulmonary hypertension, MRA and perfusion sequences making it possible to identify and differentiate between pulmonary embolism and other disease entities.(22,23) In addition, MRA allows for an in-depth evaluation of the location of the thromboembolic material, and, for surgical planning, MRA is equally as useful as are digital subtraction angiography and CT angiography.(24,25) In MRI, perfusion sequences can be quantitatively evaluated, allowing the severity of small-vessel disease to be assessed. The reduction in perfusion correlates with disease severity, and preliminary results suggest that perfusion is a sensitive surrogate for disease monitoring.(4) Structural imaging of the lung will allow the exclusion of parenchymal diseases. Measurements of blood flow and right heart pressures allow pulmonary arterial pressure and cardiac strain to be estimated, as well as facilitating the identification of concomitant valvular disease.
Patients with cystic fibrosisCystic fibrosis is an autosomal recessive disorder caused by mutations in a single gene (located on the long arm of chromosome 7) that encodes the cystic fibrosis transmembrane conductance regulator protein, which functions as an anion channel. The standard radiological tools for monitoring lung disease in patients who have cystic fibrosis are chest X-ray and HRCT, the results of which are evaluated with various scoring systems.(26) Scans obtained using HRCT provide images of lung structure with micrometer-scale resolution, and HRCT findings can also constitute useful outcome measures for studies of lung disease in cystic fibrosis patients.(27,28)
During recent years, a variety of strategies have been developed to overcome the inherent difficulties of MRI of the lung, leading to substantial improvements in temporal and spatial resolution, as evidenced in Figure 7.(8) Although the spatial resolution of MRI is lower than is that of CT, the former has the advantage of better contrast-enhanced imaging of tissues. In addition, MRI can be used in order to assess various aspects of pulmonary function, including lung perfusion,(29,30) blood flow,(31) respiratory mechanics,(32,33) and (with the administration of inhaled contrast agents) pulmonary ventilation.(34)
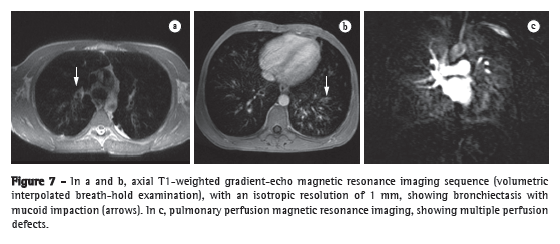
Although there are few reports in the literature, MRI is widely used as a means of screening for cystic fibrosis and surveillance of cystic fibrosis patients. Recent studies have shown that MRI is highly capable of revealing the bronchiectasis typical of cystic fibrosis, as well as mucus plugging, and that MRI has a diagnostic value equal to that of CT in grading the severity of the disease with the Bhalla or Helbich score.(35)
Patients with pneumoniaThe various features of pneumonia, such as ill-defined nodules, ground-glass opacities, and consolidations, can be easily detected and differentiated with MRI. In chest X-ray, extremely small nodules and calcifications pose great challenges because of the thickness of the slice and the low intensity of the signal. As a follow-up tool, MRI is recommended over CT, in order to avoid excessive exposure to ionizing radiation. Figure 8 shows a comparison between a chest X-ray and MRI scans of the same chest. The sensitivity of T2-weighted sequences and the potential of contrast-enhanced T1-weighted sequences can greatly facilitate the differential diagnosis.(36) In addition, incipient complications, such as pericardial effusion, pleural effusion, empyema, and lung abscess, are easily recognized on MRI scans. In immunocompromised patients, MRI is nearly as accurate as is CT for the detection of pulmonary abnormalities.(8)
Patients with interstitial lung diseaseThe use of MRI in the assessment of interstitial lung disease (ILD) has been limited by the low proton density of the lungs, resulting in low signal-to-noise ratios and signal loss due to motion artifacts (respiratory and cardiac), as well as susceptibility artifacts due to multiple air-tissue interfaces. However, evidence suggests that MRI can play a role in the assessment of disease activity in ILD, and recent technological refinements have led to a considerable improvement in the quality of MRI scans, potentially creating opportunities for the use of MRI in select patients with ILD. Typical patterns, such as reticular/reticulonodular changes, ground-glass opacities, consolidation, and honeycombing, are easily visualized with MRI.(37) However, although MRI has the advantage of avoiding radiation exposure, the accuracy of MRI is lower than is that of CT in patients with ILD. Functional imaging, using contrast-enhanced perfusion MRI and ventilation MRI with hyperpolarized gases or oxygen, can be used for the assessment of restrictive lung disease. Routine contrast-enhanced MRI allows the inflammatory activity in various types of ILD to be estimated, giving MRI a clear advantage over CT, which has limitations in differentiating between active inflammation and early-stage fibrosis.(37)
Limitations of MRI of the lungIn healthy individuals, approximately 70% of the lung consists of air-filled spaces. Because those spaces do not contain protons, which are essential to generating an image in MRI, no signal can be detected. Although the remaining 30% contains protons, either localized in the tissue or within the blood volume, the generation of signals from such components is hampered by two major factors: air and tissue have very different magnetic properties, and the countless interfaces between these two structures therefore generate significant so-called susceptibility artifacts that ultimately result in a loss of signal for fine structures such as alveolar septae; and blood flow is pulsatile, which, together with respiratory motion and cardiac contraction, poses specific methodological challenges in MRI. Overall, the lung is probably the most difficult organ to study using MRI. That is why the lung has long been regarded as a black hole on whole-body MRI scans and continues to be a blank spot on the map of the body created through the use of various MRI applications. Calcification is an important finding, and CT is superior to MRI for the visualization of calcifications and for the characterization of calcified nodules.
Despite all of the technological advancements, most MRI scanners are not capable of performing high-quality imaging studies of the chest. The gradients and sequences designed specifically by some manufacturers constitute a major limiting factor. As an example, the third Prospective Investigation of Pulmonary Embolism Diagnosis (PIOPED III) study,(38) a multicenter study evaluating the use of MRI in pulmonary embolism, demonstrated that 11-52% of the images obtained were of poor technical quality.(38) This demonstrates not only the limitations of the equipment but also those of the staff performing the examinations. The PIOPED III study produced poor results, identifying only 59 (57%) of 104 patients with pulmonary embolism. One factor might have been that the authors did not evaluate pulmonary perfusion, which has high accuracy for the diagnosis of pulmonary embolism.
Recent findings suggest that MRI produces considerable fear and distress in a significant number of patients.(39) Up to 37% of patients who undergo MRI experience moderate to severe fear and anxiety, and 5-10% of patients cannot complete the MRI session because of claustrophobia.(39) Patient cooperation is a necessary condition for the examination because motion artifacts generally degrade the quality and diagnostic value of MRI. Prolonged scanning with additional sequences, sedation, or general anesthesia is required in order to complete the procedure in claustrophobic patients. This can result in a waste of valuable scan time and increased scanning costs.(39)
The cost of imaging studies is an important consideration. Because of the complexity of the imaging system, together with the intrinsic maintenance costs, MRI is inevitably a more expensive test than is CT. However, MRI is less expensive than is PET/CT, because the PET/CT machinery has many more components than does an MRI scanner and because of the costs of the radiopharmaceuticals required during PET/CT. In addition, MRI is a safer modality than is PET/CT, because of the differences in the imaging system itself. The powerful magnetic field and radiofrequency energy of MRI have not been shown to cause cancer or fetal abnormalities, unlike the ionizing radiation used in CT. It is of note that, although X-rays are known to cause cancer, the exact risk of cancer from receiving CT scans, even multiple CT scans, remains unknown.(40)
Final considerationsIn conclusion, with its rapid development in recent years, MRI of the lung is now truly at the threshold of broad clinical application. Disease of the airways and vasculature, as well as nodules and lung cancer, now constitute a major focus. Because MRI offers a number of advantages when compared with conventional nuclear medicine techniques, it is challenging multidetector CT in many applications. The fact that it does not involve the use of ionizing radiation puts MRI of the chest in a front-line position for all cross-sectional imaging studies in children. The unique combination of structural and functional information makes MRI attractive for use in all diseases in which the choices among innovative and expensive treatment options will actually require and benefit from an increased number of measurable parameters.
References1. Webb WR. Magnetic resonance imaging of the mediastinum, hila, and lungs. J Thorac Imaging. 1985;1(1):65-73.
2. Kauczor HU, Kreitner KF. MRI of the pulmonary parenchyma. Eur Radiol. 1999;9(9):1755-64.
3. Mendonça-Dias MH, Gaggelli E, Lauterbur PC. Paramagnetic contrast agents in nuclear magnetic resonance medical imaging. Semin Nucl Med. 1983;13(4):364-76.
4. Ley S, Kauczor HU. MR imaging/magnetic resonance angiography of the pulmonary arteries and pulmonary thromboembolic disease. Magn Reson Imaging Clin N Am. 2008;16(2):263-73, ix.
5. Ley-Zaporozhan J, Puderbach M, Kauczor HU. MR for the evaluation of obstructive pulmonary disease. Magn Reson Imaging Clin N Am.
2008;16(2):291-308, ix.
6. Biederer J, Hintze C, Fabel M. MRI of pulmonary nodules: technique and diagnostic value. Cancer Imaging. 2008;8:125-30.
7. Ohno Y, Koyama H, Nogami M, Takenaka D, Yoshikawa T, Yoshimura M, et al. STIR turbo SE MR imaging vs. coregistered FDG-PET/CT: quantitative and qualitative assessment of N-stage in non-small-cell lung cancer patients. J Magn Reson Imaging. 2007;26(4):1071-80.
8. Eibel R, Herzog P, Dietrich O, Rieger CT, Ostermann H, Reiser MF, et al. Pulmonary abnormalities in immunocompromised patients: comparative detection with parallel acquisition MR imaging and thin-section helical CT. Radiology. 2006;241(3):880-91.
9. Lillington GA. Disease-a-Month. St Louis: Mosby-Year Book; 1991. p. 271-318.
10. Cronin P, Dwamena BA, Kelly AM, Carlos RC. Solitary pulmonary nodules: meta-analytic comparison of cross-sectional imaging modalities for diagnosis of malignancy. Radiology. 2008;246(3):772-82.
11. Koyama H, Ohno Y, Kono A, Takenaka D, Maniwa Y, Nishimura Y, et al. Quantitative and qualitative assessment of non-contrast-enhanced pulmonary MR imaging for management of pulmonary nodules in 161 subjects. Eur Radiol. 2008;18(10):2120-31.
12. Ohno Y, Koyama H, Dinkel J, Hintze C. Lung Cancer. In: Kauczor HU, editor. MRI of the lung. Heidelberg: Springer Berlin Heidelberg; 2009. p. 179-216.
13. Schmidt GP, Baur-Melnyk A, Herzog P, Schmid R, Tiling R, Schmidt M,
et al. High-resolution whole-body magnetic resonance image tumor staging with the use of parallel imaging versus dual-modality positron emission tomography-computed tomography: experience on a 32-channel system. Invest Radiol. 2005;40(12):743-53.
14. Ohno Y, Koyama H, Onishi Y, Takenaka D, Nogami M, Yoshikawa T, et al. Non-small cell lung cancer: whole-body MR examination for M-stage assessment--utility for whole-body diffusion-weighted imaging compared with integrated FDG PET/CT. Radiology. 2008;248(2):643-54.
15. Yung GL, Fedullo PF. Pulmonary Thromboembolic Disease. In: Fishman A, Elias JA, editors. Fishman's Pulmonary Diseases and Disorders. New York: McGraw-Hill Medical Pub Division; 2008. p. 1297-330.
16. Castañer E, Gallardo X, Ballesteros E, Andreu M, Pallardó Y, Mata JM, et al. CT diagnosis of chronic pulmonary thromboembolism. Radiographics. 2009;29(1):31-50; discussion 50-3.
17. Fink C, Ley S, Kroeker R, Requardt M, Kauczor HU, Bock M. Time-resolved contrast-enhanced three-dimensional magnetic resonance angiography of the chest: combination of parallel imaging with view sharing (TREAT). Invest Radiol. 2005;40(1):40-8.
18. Kluge A, Luboldt W, Bachmann G. Acute pulmonary embolism to the subsegmental level: diagnostic accuracy of three MRI techniques compared with 16-MDCT. AJR Am J Roentgenol. 2006;187(1):W7-14.
19. Ohno Y, Higashino T, Takenaka D, Sugimoto K, Yoshikawa T, Kawai H, et al. MR angiography with sensitivity encoding (SENSE) for suspected pulmonary embolism: comparison with MDCT and ventilation-perfusion scintigraphy. AJR Am J Roentgenol. 2004;183(1):91-8.
20. Stein PD, Woodard PK, Hull RD, Kayali F, Weg JG, Olson RE, et al. Gadolinium-enhanced magnetic resonance angiography for detection of acute pulmonary embolism: an in-depth review. Chest. 2003;124(6):2324-8.
21. Oudkerk M, van Beek EJ, Wielopolski P, van Ooijen PM, Brouwers-Kuyper EM, Bongaerts AH, et al. Comparison of contrast-enhanced magnetic resonance angiography and conventional pulmonary angiography for the diagnosis of pulmonary embolism: a prospective study. Lancet. 2002;359(9318):1643-7.
22. Ley S, Fink C, Zaporozhan J, Borst MM, Meyer FJ, Puderbach M, et al. Value of high spatial and high temporal resolution magnetic resonance angiography for differentiation between idiopathic and thromboembolic pulmonary hypertension: initial results. Eur Radiol. 2005;15(11):2256-63.
23. Nikolaou K, Schoenberg SO, Attenberger U, Scheidler J, Dietrich O, Kuehn B, et al. Pulmonary arterial hypertension: diagnosis with fast perfusion MR imaging and high-spatial-resolution MR angiography--preliminary experience. Radiology. 2005;236(2):694-703.
24. Kreitner KF, Ley S, Kauczor HU, Mayer E, Kramm T, Pitton MB, et al. Chronic thromboembolic pulmonary hypertension: pre- and postoperative assessment with breath-hold MR imaging techniques. Radiology. 2004;232(2):535-43.
25. Kreitner KF, Kunz RP, Ley S, Oberholzer K, Neeb D, Gast KK, et al. Chronic thromboembolic pulmonary hypertension - assessment by magnetic resonance imaging. Eur Radiol. 2007;17(1):11-21.
26. Bhalla M, Turcios N, Aponte V, Jenkins M, Leitman BS, McCauley DI, et al. Cystic fibrosis: scoring system with thin-section CT. Radiology. 1991;179(3):783-8.
27. Brody AS, Molina PL, Klein JS, Rothman BS, Ramagopal M, Swartz DR. High-resolution computed tomography of the chest in children with cystic fibrosis: support for use as an outcome surrogate. Pediatr Radiol. 1999;29(10):731-5.
28. Robinson TE. High-resolution CT scanning: potential outcome measure. Curr Opin Pulm Med. 2004;10(6):537-41.
29. Levin DL, Chen Q, Zhang M, Edelman RR, Hatabu H. Evaluation of regional pulmonary perfusion using ultrafast magnetic resonance imaging. Magn Reson Med. 2001;46(1):166-71.
30. Sridharan S, Derrick G, Deanfield J, Taylor AM. Assessment of differential branch pulmonary blood flow: a comparative study of phase contrast magnetic resonance imaging and radionuclide lung perfusion imaging. Heart. 2006;92(7):963-8.
31. Levin DL, Hatabu H. MR evaluation of pulmonary blood flow. J Thorac Imaging. 2004;19(4):241-9.
32. Chen Q, Mai VM, Bankier AA, Napadow VJ, Gilbert RJ, Edelman RR. Ultrafast MR grid-tagging sequence for assessment of local mechanical properties of the lungs. Magn Reson Med. 2001;45(1):24-8.
33. Sundaram TA, Gee JC. Towards a model of lung biomechanics: pulmonary kinematics via registration of serial lung images. Med Image Anal. 2005;9(6):524-37.
34. Middleton H, Black RD, Saam B, Cates GD, Cofer GP, Guenther R, et al. MR imaging with hyperpolarized 3He gas. Magn Reson Med. 1995;33(2):271-5.
35. Puderbach M, Eichinger M, Haeselbarth J, Ley S, Kopp-Schneider A, Tuengerthal S, et al. Assessment of morphological MRI for pulmonary changes in cystic fibrosis (CF) patients: comparison to thin-section CT and chest x-ray. Invest Radiol. 2007;42(10):715-25.
36. Eibel R. Pneumonia. In: Kauczor HU, editor. MRI of the lung. Heidelberg: Springer Berlin Heidelberg; 2009. p. 255-77.
37. Marten K, Molinari F. Interstitial lung disease. In: Kauczor HU, editor. MRI of the lung. Heidelberg: Springer Berlin Heidelberg; 2009. p. 277-91.
38. Stein PD, Chenevert TL, Fowler SE, Goodman LR, Gottschalk A, Hales CA, et al. Gadolinium-enhanced magnetic resonance angiography for pulmonary embolism: a multicenter prospective study (PIOPED III). Ann Intern Med. 2010;152(7):434-43, W142-3.
39. Hollenhorst J, Münte S, Friedrich L, Heine J, Leuwer M, Becker H, et al. Using intranasal midazolam spray to prevent claustrophobia induced by MR imaging. AJR Am J Roentgenol. 2001;176(4):865-8.
40. Santos MK, Elias J Jr, Mauad FM, Muglia VF, Trad CS. Magnetic resonance imaging of the chest: current and new applications, with an emphasis on pulmonology. J Bras Pneumol. 2011;37(2):242-58.
41. Huda W. Radiation doses and risks in chest computed tomography examinations. Proc Am Thorac Soc. 2007;4(4):316-20.
Study carried out at the Moinhos de Vento Hospital, Porto Alegre, Brazil.
Correspondence to: Bruno Hochhegger. Rua João Alfredo, 558/301, Cidade Baixa, CEP 90050-230, Porto Alegre, RS, Brasil.
Financial support: None.
Submitted: 1 November 2011. Accepted, after review: 3 November 2011.
** A versão completa em português deste artigo está disponível em www.jornaldepneumologia.com.brReview
About the authorsBruno Hochhegger
Chest Radiologist. Santa Casa Sisters of Mercy Hospital Complex; Professor of Radiology, Federal University of Health Sciences of Porto Alegre, Porto Alegre, Brazil.
Edson Marchiori
Professor of Radiology. Federal University of Rio de Janeiro, Rio de Janeiro, Brazil.
Klaus Irion
Chest Radiologist. Liverpool Heart and Chest Hospital, Liverpool, UK.
Arthur Soares Souza Junior
Professor of Radiology. São José do Rio Preto School of Medicine, São
José do Rio Preto, Brazil.
Jackson Volkart
Chest Radiologist. Moinhos de Vento Hospital, Porto Alegre, Brazil.
Adalberto Sperb Rubin
Pulmonologist. Santa Casa Sisters of Mercy Hospital Complex, Porto Alegre, Brazil.