ABSTRACT
Objective: To evaluate the effects of passive inhalation of cigarette smoke on the respiratory system of guinea pigs. Methods: Male guinea pigs were divided into two groups: control and passive smoking, the latter being exposed to the smoke of ten cigarettes for 20 min in the morning, afternoon and evening (30 cigarettes/day) for five days. After that period, inflammatory parameters were studied by quantifying mesenteric mast cell degranulation, as well as oxidative stress, in BAL fluid. In addition, we determined MIP, MEP, and mucociliary transport (in vivo), as well as tracheal contractility response (in vitro). Results: In comparison with the control group, the passive smoking group showed a significant increase in mast cell degranulation (19.75 ± 3.77% vs. 42.53 ± 0.42%; p < 0.001) and in the levels of reduced glutathione (293.9 ± 19.21 vs. 723.7 ± 67.43 nM/g of tissue; p < 0.05); as well as a significant reduction in mucociliary clearance (p < 0.05), which caused significant changes in pulmonary function (in MIP and MEP; p < 0.05 for both) and airway hyperreactivity. Conclusions: Passive inhalation of cigarette smoke caused significant increases in mast cell degranulation and oxidative stress. This inflammatory process seems to influence the decrease in mucociliary transport and to cause changes in pulmonary function, leading to tracheal hyperreactivity.
Keywords:
Inflammation; Inhalation exposure; Tobacco smoke pollution.
RESUMO
Objetivo: Investigar a correlação entre a carga viral do vírus sincicial respiratório e o tempo de internação hospitalar em lactentes com episódios de sibilância aguda. Métodos: Este foi um estudo transversal de dois anos envolvendo lactentes de até 12 meses de idade com bronquiolite no momento da internação em um hospital terciário. Para a identificação dos vírus respiratórios foram coletadas secreções nasofaríngeas. As amostras foram analisadas (por todo o período do estudo) por imunofluorescência direta e (no segundo ano do estudo) por PCR quantitativa em tempo real para três vírus humanos (rinovírus, vírus sincicial respiratório e metapneumovírus). Resultados: Das 110 amostras avaliadas por imunofluorescência direta, 56 (50,9%) foram positivas para um único vírus, e 16 (14,5%) foram positivas para dois ou mais vírus. Nessas 72 amostras, o vírus mais prevalente foi o vírus sincicial respiratório, seguido por influenza. Das 56 amostras avaliadas por PCR quantitativa em tempo real, 24 (42,8%) foram positivas para um único vírus, e 1 (1,7%) foi positiva para dois vírus. Nessas 25 amostras, o vírus mais prevalente foi o vírus sincicial respiratório, seguido por rinovírus humano. A coinfecção não influenciou o tempo de internação ou outros desfechos. Além disso, não houve associação entre a carga viral de vírus sincicial respiratório e o tempo de internação. Conclusões: A coinfecção e a carga viral do vírus sincicial respiratório não parecem influenciar os desfechos em lactentes com bronquiolite aguda.
Palavras-chave:
Inflamação; Exposição por inalação; Poluição por fumaça de tabaco.
INTRODUCTIONSmoking is a risk factor for the leading causes of death worldwide, including cardiac and pulmonary diseases. (1,2) There are no safe levels of exposure to cigarettes. Passive or active smoking is directly related to irritation, inflam-mation, and changes in lung function within the first few hours of exposure.(3,4)
Airway contact with cigarette smoke induces changes in the respiratory system, such as mucus hypersecretion, def-icit in mucociliary transport, tracheobronchial tree defects, small airway restriction accompanied by increased closing capacity, and a trend toward changes in the ventilation-perfusion ratio.(5)
A passive smoker is an individual who inhales environmental cigarette smoke. The immediate harmful effects of such exposure include irritation of the eyes, nose and throat, as well as increased respiratory and heart rates.(6) There is also an increase in inflammatory cytokine levels within the first few hours of inhalation, especially in men.(3)
The concern regarding passive inhalation of cigarette smoke is recent, having begun in the early 1980s. According to the Brazilian National Health Oversight Agency, approximately two thirds of the smoke produced by cigarettes (passive smoking, secondary exposure, passive inhalation, or involuntary smoking) is released into the ambient air through the lit end of the product. (7) In the USA, 3,000 lung cancer deaths per year are estimated to have been caused by passive inhalation of cigarette smoke.(8) In Brazil, the National Health Survey conducted in 2013 points out that the proportion of people aged 18 years or older who were exposed to passive smoking was 10.7% in the home and 13.5% in the indoor workplace. Regarding gender, that proportion is higher among women in the home (11.7%) and men in the workplace (16.9%).(9)
Some studies have pointed out that the harmful effects of passive inhalation of cigarette smoke may begin in child-hood, causing cough,(10) endothelial dysfunction,(11) and prolonged expiratory apnea.(12)
Currently, the incidence of death and disease caused by cigarette smoking remains high. However, there have been few studies reporting the possible structural and functional changes in the respiratory system in passive smok-ers. This aroused our interest in developing this study. We hope to use the study findings to alert the population to and raise its awareness about the prevention of diseases caused by smoking, in order to contribute to improving public health in general.
Therefore, the present study aimed at evaluating structural and functional aspects of the respiratory system of guin-ea pigs after passive inhalation of cigarette smoke.
METHODSThis was an experimental longitudinal exploratory study with quantitative analysis of results. We used male guinea pigs (Cavia porcellus; 5-8 per group) obtained from the animal facilities of the Estácio University Center of Ceará, located in the city of Fortaleza, Brazil. The mean body weight of the animals was 321.00 ± 6.72 g at the beginning of the experiments. All animals were handled in accordance with the Brazilian College for Animal Experimentation's guidelines for animal care and welfare. The study was approved by the Animal Research Ethics Committee of the Federal University of Ceará (Protocol no. 052/10).
The experiments were performed in the Laboratory of Biophysics, Physiology and Pharmacology of the Centro Uni-versitário Estácio do Ceará (Estácio University Center of Ceará), in cooperation with the Department of Physiology and Pharmacology of the Federal University of Ceará, also located in the city of Fortaleza.
Cigarette smoke inhalationThe protocol consisted of inhalation of smoke from ten commercial filter cigarettes (Derby Autêntico; Souza Cruz S.A., Rio de Janeiro, Brazil), each containing 8 mg of tar, 0.7 mg of nicotine, and 7 mg of carbon monoxide. Those cigarettes were lit concomitantly for 20 min in the morning, afternoon, and evening, adding up to 30 cigarettes/day, for 5 days.(13,14) During inhalation, the animals were placed into acrylic boxes (whole body; n = 3 per box) measur-ing 30.0 × 16.6 × 19.8 cm.(15) Each box had a removable upper lid for introduction of the animals and two holes on its side walls: one for air drainage, allowing the smoke to escape; and one for placing the cigarettes (cigarette smoke was introduced into the acrylic box passively). Control animals did not inhale any type of toxic substance, and they were placed into control group acrylic boxes (whole body; n = 3 per box) to simulate the same conditions as those of the cigarette smoke inhalation groups.
For the purposes of this study, two groups were formed: control, consisting of guinea pigs that did not inhale any toxic substance and were killed after 25 days; and passive smoking, consisting of guinea pigs that were killed 25 days after completing the process of passive inhalation of cigarette smoke (Figure 1).
Analysis of the inflammatory process on the basis of mast cell degranulation countsAfter anesthesia (urethane, 1.2g/kg, i.p.), the mesentery was removed and a wire was carefully inserted into the small intestine, which was then given a circular shape. Subsequently, the small intestine was transferred to a Petri dish containing a toluidine blue solution (toluidine blue dissolved in 70% alcohol at a concentration of 0.1g/L and diluted with 1% NaCl at a ratio of 1:10, resulting in a concentration of 0.01 g/L) for staining at room temperature. The preparation was then washed with Tyrode's solution (136 mM of NaCl; 5 mM of KCI; 0.98 mM of MgCl2; 0.36 mM of NaH2PO4; 11.9 mM of NaHCO3; 2 mM of CaCl2; and 5.5 mM of glucose) and left to dry on a glass slide (for 10 min) at room temperature. After drying and excess tissue removal, 100 cells were counted in different fields by optical mi-croscopy (magnification, ×200), and, on that basis, the percentage of mast cell degranulation was determined.
Assessment of oxidative stressOxidative stress was measured indirectly by quantification of nitrite and reduced glutathione (GSH) in BAL fluid. To that end, the lungs were initially filled with 5 mL of saline solution at 37ºC, which was instilled into the tracheal tube using a syringe. After a 3-min period, the instilled fluid was slowly recovered by aspiration. That procedure was repeated with another 5 mL of saline solution. The material was stored in a freezer (−70ºC).(16)
Determination of nitriteAssays were prepared with 100 µL of Griess reagent-0.1% N-(1-naphthyl)ethylenediamine in water; and 1% sul-fanilamide in 5% phosphoric acid-and 100 µL of the (centrifuged) supernatant of the 20% BAL fluid homogenate from the guinea pigs or 100 µl of various concentrations of the standards. Blanks were prepared with 100 µL of Griess reagent and 100 µL of saline solution (0.9% NaCl). Absorbance was measured at 560 nm using a plate reader.(17) Results are expressed as µM/g of tissue.
Determination of GSHThe reagent was prepared using 0.02 M of EDTA and 50% trichloroacetic acid. After this process, centrifugation was performed (5,000 rpm for 15 min at 4°C). Subsequently, the supernatant was collected and homogenized. The sam-ples were mixed with 0.4 M Tris-HCl buffer (pH = 8) and 0.01 M of 5.5-dithiobis-2-nitrobenzoic acid. The material was kept cooled throughout the assay. The GSH activity was measured at 412 nm using a plate reader. Results are ex-pressed as ng/g of tissue.
Measurement of mucociliary transportInitially, the guinea pigs were anesthetized with urethane (1.2 g/kg, i.p.) and fixed horizontally in the supine posi-tion. Subsequently, 2 µL of a 0.3 g/mL gelatin solution containing 0.5% Evans blue dye was injected into their trache-as with a microsyringe. Two minutes later, the tracheas were opened, and mucociliary transport was measured, from the injection point, by using a caliper.(15,18)
Measurement of pulmonary pressuresThe animals were anesthetized (urethane, 1.2 g/kg, i.p.). Subsequently, the trachea was cannulated and connected in a closed system to a pressure transducer, at the end of inhalation, not allowing airflow leaks. The connection was maintained until the animal had intercostal retraction (approximately 20 seconds). Three MIP and MEP measurements were taken in each animal, and the time interval between measurements depended on normalization of the respirato-ry pattern and rhythm.(19) The ventilatory parameters were recorded by PowerLab/8sp (ADInstruments Pty Ltd., Bella Vista, Australia) data acquisition system.
Isometric recordings of the tracheal ringsAfter anesthesia (urethane, 1.2 g/kg, i.p.) and subsequent euthanasia by exsanguination (through the left carotid artery), a ventral midline incision was made and the trachea was rapidly excised in one single segment of approxi-mately 10-12 mm.(19) The excised segment was then rapidly transported to a Petri dish containing Krebs-Henseleit solution (in nmol/L: 118 NaCl; 4.7 KCI; 2.5 CaCl2; 1.2 MgSO4; 25.0 NaHCO3; 1.2 KH2PO4; and 10 glucose). After re-moval of adjacent tissues, the trachea was cut into four ring-shaped segments, which were transferred to individual organ bath chambers containing 5 mL of Krebs-Henseleit solution that was continuously aerated with carbogen ((95% O2 and 5% CO2); the pH of the solution was manually adjusted to 7.4 at a temperature of 37°C, which was kept con-stant through water circulation from a pumped water bath. The lumen of the tracheal rings was crossed with two pieces made of thin stainless steel, which were tied to two points: one fixed in the chamber; and one connected to a force transducer (ML870B60/C-V; ADInstruments) suitable for recording isometric contractions. The signals generated by the force transducer were recorded by a computerized data acquisition system (PowerLabT 8/30; ADInstruments). The tension applied to each tracheal segment was set at 1 gf. The equilibration period was 1 h, and the incubation liquid was changed every 15 min.(20) After the stabilization period, concentration-effect curves were constructed for potassium (10-120 mM) and for carbachol (0.001-10 µM), with a 5 min-interval at each concentration. After comple-tion of the protocol, the tracheas were removed from the incubation liquid and left to dry at room temperature (ap-proximately 25°C) for 2 h. Subsequently, each tissue was weighed.
Data analysisResults are expressed as mean ± standard error of the mean, and the number of experimental observations is not-ed in parentheses (n). For group comparison, we used one-way or two-way ANOVA, as well as the Student's t-test, Holm-Sidak's test, the Kruskal-Wallis test, and the Mann-Whitney test, according to the normality test. For all tests, values of p < 0.05 were considered statistically significant.
RESULTSMast cell degranulationMesenteric mast cell degranulation in the guinea pigs was 19.75 ± 3.77% (n = 5). However, in the guinea pigs that inhaled cigarette smoke over a short period of time (25 days), mast cell degranulation was 42.53 ± 0.42% (n = 5), being significantly higher than that observed in the control group (p < 0.001; Student's t-test; Figure 2).
Nitrite and GSH levelsDetermination of nitrite (control group = 0.073 ± 0.007 µM/g of tissue vs. passive smoking group = 0.065 ± 0.004 µM/g of tissue) and GSH (control group = 293.9 ± 19.21 nM/g of tissue vs. passive smoking group = 723.7 ± 67.43 nM/g of tissue) in the BAL fluid from the guinea pigs revealed a significant difference (p < 0.05; Student's t-test) only in the GSH levels (Figure 3).
Mucociliary transport and pulmonary pressuresAnalysis of mucociliary transport revealed that, in the control group, the distance traveled was 0.65 ± 0.08 cm; however, that distance was significantly reduced (p < 0.05; ANOVA followed by the Kruskal-Wallis post-test) to 0.30 ± 0.03 cm in the passive smoking group (n = 6; Figure 4A).
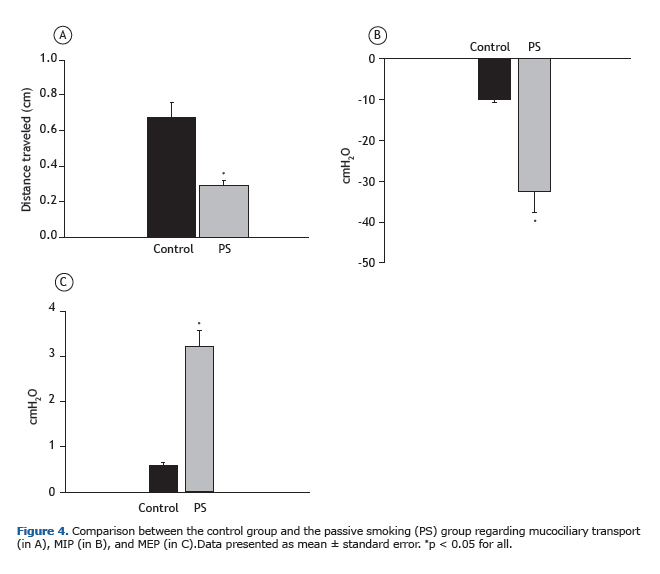
The mean MIP was −9.93 ± 0.94 cmH2O in the control group (n = 5) and −40.44 ± 9.26 cmH2O in the passive smoking group (n = 6); therefore, the latter group showed a significant decrease (p < 0.05; ANOVA followed by Holm-Sidak's post-test) as compared with the former (Figure 4B).
The mean MEP was 0.58 ± 0.05 cmH2O in the control group (n = 6) and 3.60 ± 0.60 cmH2O in the passive smoking group (n = 6); therefore, the latter group showed a significant increase (p < 0.05; ANOVA followed by Holm-Sidak's post-test) as compared with the former (Figure 4C).
Tracheal contractilityAdding increasing cumulative concentrations of K+ (10-120 mM) in the control group (n = 6) produced a contractile response with an amplitude of 0.05 ± 0.00 gf/mg of tissue. Comparatively, the passive smoking group (n = 8) showed a significantly increased (p < 0.05; two-way ANOVA followed by Holm-Sidak's post-test) contractile re-sponse, with a mean value of 0.10 ± 0.01 gf/mg of tissue (Figure 5A).
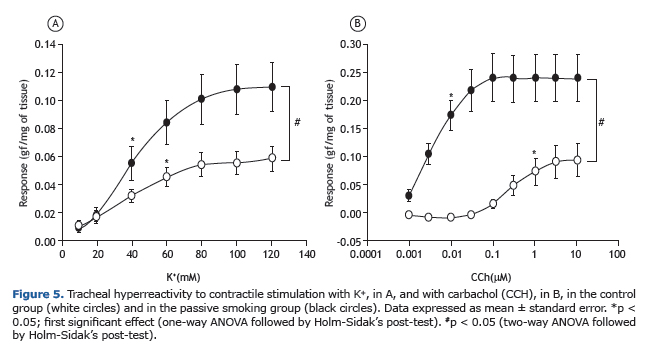
Concentration-effect curves were also constructed to determine the contractile response to carbachol (0.001-10 µM): the animals in the control group (n = 6) showed a mean value of 0.09 ± 0.02 gf/mg of tissue; comparatively, the animals in the passive smoking group (n = 8) showed a significantly increased (p < 0.05; two-way ANOVA followed by Holm-Sidak's post-test) contractile response (0.23 ± 0.03 gf/mg of tissue; Figure 5B).
DISCUSSIONThe findings of the present study reveal that passive inhalation of cigarette smoke over a short period of time pro-duces structural and functional changes in the respiratory system. Such effects were proven by evidence of a deficit in lung tissue; an increase in inflammatory cells, leading to mucus accumulation; and pulmonary function impairment caused by tracheal hyperreactivity. Although some inhalation models(13,14,21,22) have reported the damages caused by constant and prolonged use of cigarettes, the relevance of the present study lies in reporting the changes caused by passive inhalation of cigarette smoke over a short period of time (5 days), with a change in the amount and dura-tion of exposure to cigarette smoke as compared with previous studies. (23-25) In their study, Hernandez et al.(23) also opted to analyze the effects of passive inhalation of cigarette smoke over a short period of time. To that end, the animals were submitted to a protocol consisting of three 10-min exposures to smoke from one cigarette, separated by 30-min intervals, each day, for 4 days, and it was found that exposure of guinea pigs to cigarette smoke produced airway hyperreactivity to histamine and recruitment of inflammatory cells.
It is of note that, in recent years, the understanding of the pathophysiological mechanisms related to passive inha-lation of cigarette smoke has enabled increasingly specific approaches, with the use of concise and reproducible methods to investigate the contractile and inflammatory repercussions of this inhalation process.
The animals that inhaled cigarette smoke exhibited destruction of lung tissue architecture, accompanied by migra-tion of proinflammatory cells (data not shown), which corroborates other experimental models of cigarette smoke inhalation.(23-27) Such changes were pointed out by the study of Banerjee et al.,(28) in which the guinea pigs that in-haled cigarette smoke exhibited inflammation and apoptosis, which leads to destruction of alveolar membranes and septal cells, causing pulmonary airspace enlargement, and such enlargement may have influenced the changes in pulmonary pressures in the present study. Page et al.(29) add that, after exposure of airway sensory nerves to ciga-rette smoke, there is damage caused by cytotoxic mediators to the ciliary epithelium layer, increased mucus secre-tion, hyperresponsiveness, and vasodilatation, findings that are in agreement with those of the present study.
In this sense, Valença & Porto(21) highlighted the participation of macrophages in the destruction of extracellular lung matrix in animals exposed to cigarette smoke over different periods of time; in contrast, in animals exposed to room air (control group), the alveoli were preserved and there were few alveolar macrophages. The study by Zhong et al.,(30) in which guinea pigs were exposed to inhalation of smoke from 10 cigarettes for 20 min, twice a day, for 14 days, reported that the animals exposed to cigarette smoke showed increased proinflammatory cytokine levels (TNF-α and IL-8), as well as an increase in inflammatory cells and tracheal thickness.
Another important harmful effect observed in the present study was the decrease in mucociliary transport in the guinea pigs submitted to passive inhalation of cigarette smoke, a finding confirmed by Furtado(5) when he states that smoking leads to a decrease in mucociliary transport because of the many toxic substances found in the cigarette that exhibit ciliostatic and ciliotoxic properties. Vasconcelos et al.(15) also observed a deficit in mucociliary transport in guinea pigs that mimicked asthma symptoms, corroborating the present study when they state that inflammatory diseases cause changes in ciliary function and in the amount of mucus secreted, which directly influences the protec-tion of the respiratory system.
Therefore, to protect itself, the organism has an intracellular defense system that can act in two ways: either by re-ducing the toxic substances in the organism before they cause injury, through the activity of antioxidants, such as GSH, superoxide dismutase, catalase, and vitamin E; or by inhibiting the injury with ascorbic acid, glutathione reduc-tase, glutathione peroxidase, etc.(31) Indirectly, measurement of antioxidant enzyme activity and determination of the concentration of tripeptides are the most widely used methods for the assessment of oxidative stress.(32) That same group of authors(32) points out the important antioxidant effect of GSH on the respiratory tract, an effect that can re-duce oxidative stress.
The levels of GSH, one of the major antioxidant enzymes in the respiratory tract, were significantly higher in the passive smoking group than in the control group, demonstrating an adaptive response of the organism to an injury, but there were no changes in the nitrite levels. MacNee & Rahman(33) add that, initially, there is a decrease in GSH levels, but, over time, these levels are increased to protect against the toxic damage caused by cigarette smoke inhalation. However, this increase in GSH levels may not be sufficient to neutralize the excessive oxidant burden during acute smoking.
In this sense, several studies support the relationship between cigarette smoke inhalation and the production of oxidative stress,(33-35) because cigarettes are known to be a potential inducer of adverse effects in biological struc-tures and in tissues, as well as influencing weight loss, inflammatory cell increase, and muscle fatigue. (26,35) Carlos et al.,(26) in a study in which male (C57BL/6) mice were exposed to smoke from 12 cigarettes a day for 7, 15, 30, 45, and 60 days, identified that the oxidative stress induced by cigarette smoke caused changes in the lungs and in the diaphragm. Those authors added that the oxidative stress caused by exposure to cigarette smoke occurs first in the skeletal muscle and subsequently in the lung tissue. Regarding deficits in muscle strength, Camargo Filho et al.(36) point out that cigarette smoke can lead to muscle atrophy, which is a way for the organism to adapt to oxygen limita-tion, causing loss of activity and disorganization of the muscle fibers.
The animals that inhaled cigarette smoke also exhibited tracheal hyperreactivity to electromechanical contractile stimulation (K+) and to pharmacomechanical contractile stimulation (carcachol), possibly because of reduced epithe-lial protection after cigarette smoke inhalation.(37) The results presented here support the hypothesis that the process of cigarette smoke inhalation produces increased responses in voltage-operated Ca2+ channels and in receptor-operated Ca2+ channels.(38,39)
The hyperreactivity reported in the present model of passive inhalation of cigarette smoke is similar to that found in models of asthma and chronic inflammatory bronchoconstrictive disease.(22,23,37)
James et al.(40) pointed out that cigarette smoke inhalation produces harmful effects in the respiratory system of guinea pigs, such as increased airway resistance; however, such effects did not produce an increase in airway wall thickness. Omini et al.(41) add that passive exposure to cigarette smoke produces a significant increase in the number of macrophages and eosinophils in the BAL fluid, which further corroborates the tracheal hyperreactivity, but no mucus hypersecretion.
Hernandez et al.(23) conducted a study to investigate the ability of a mucoactive compound (CO 1408) to modulate the development of airway hyperreactivity induced by passive inhalation of cigarette smoke over a short period of time. Those authors found that passive inhalation of cigarette smoke causes airway hyperreactivity associated with an increase in inflammatory cells, and that the CO 1408 compound was able to reverse these harmful effects.
The limitations of the present study include the fact that the experimental model of passive inhalation of cigarette smoke was performed over a short period of time and the long-term effects of inhalation were not assessed. There-fore, new approaches that address these issues, as well as the insertion of forms of prevention and treatment, should be attempted.
In conclusion, the protocol of passive inhalation of cigarette smoke used in the present study was effective, because it mimicked structural and functional changes in the guinea pig respiratory system that characterize the damages caused by cigarette smoke.
ACKNOWLEDGMENTSWe would like to thank Professor Rodrigo José Bezerra Siqueira for his comments during the writing of the manu-script. We are also grateful to the Department of Physiology and Pharmacology of the Federal University of Ceará for the structural support.
REFERENCES1. Burns DM. Epidemiology of smoking-induced cardiovascular disease. Prog Cardiovasc Dis. 2003;46;(1):11-29. http://dx.doi.org/10.1016/S0033-0620(03)00079-3
2. Almeida AF, Mussi FC. Smoking: knowledge, attitudes, habits and degree of dependence of young adults in Salvador [Article in Portuguese]. Rev Esc Enferm USP. 2006;40(4):456-63. http://dx.doi.org/10.1590/S0080-62342006000400002
3. Flouris AD, Metsios GS, Carrillo AE, Jamurtas AZ, Gourgoulianis K, Kiropoulos T, et al. Acute and short-term effects of secondhand smoke on lung function and cytokine production. Am J Respir Crit Care Med. 2009;179(11):1029-33. http://dx.doi.org/10.1164/rccm.200812-1920OC
4. Rufino R, Costa CH. Etiopathogenesis of COPD [Article in Portuguese]. Pulmao RJ. 2013;22(2):9-14.
5. Furtado RD. Smoking and anesthetic implications [Article in Portuguese]. Rev Bras Anestesiol. 2002;52(3):354-67.
6. Coelho SA, Rocha SA, Jong LC. Consequências do tabagismo passivo em crianças. Cien Cuid Saude. 2012;11(2):294-301. http://dx.doi.org/10.4025/cienccuidsaude.v11i2.10281
7. Brasil. Ministério da Saúde. Agência Nacional de Vigilância Sanitária. Gerência de Produtos Derivados do Tabaco. A ANVISA na redução à exposição involuntária à fumaça do tabaco. Brasília: GPDTA/ANVISA; 2009.
8. Office on Smoking and Health (US). The Health Consequences of Involuntary Exposure to Tobacco Smoke: A Report of the Surgeon General. Atlanta (GA): Centers for Disease Control and Prevention (US); 2006. Available from: http://www.ncbi.nlm.nih.gov/books/NBK44324/
9. Malta DC, Andrade SS, Stopa SR, Pereira CA, Szwarcwald CL, Silva-Júnior JB, et al. Brazilian lifestyles: National Health Survey results, 2013. Epidemiol Serv Saude. 2015;24(2): 217-26. http://dx.doi.org/10.5123/S1679-49742015000200004
10. Ekwo EE, Weinberger MM, Lachenbruch PA, Huntley WH. Relationship of parental smoking and gas cooking to respiratory disease in children. Chest. 1983;84(6):662-8. http://dx.doi.org/10.1378/chest.84.6.662
11. Hashemi M, Afshani MR, Mansourian M, Poursafa P, Kelishadi R. Association of particulate air pollution and secondhand smoke on endothelium-dependent brachial artery dilation in healthy children. J Res Med Sci. 2012;17(4):317-21.
12. Mutoh T, Joad JP, Bonham AC. Chronic passive cigarette smoke exposure augments bronchopulmonary C-fibre inputs to nucleus tractus solitarii neurones and reflex output in young guinea-pigs. J Physiol. 2000;523 Pt 1:223-33. http://dx.doi.org/10.1111/j.1469-7793.2000.00223.x
13. Cendon SP, Battlehner C, Lorenzi Filho G, Dohlnikoff M, Pereira PM, Conceição GM, et al. Pulmonary emphysema induced by passive smoking: an experimental study in rats. Braz J Med Biol Res. 1997;30(10):1241-7. http://dx.doi.org/10.1590/S0100-879X1997001000017
14. Duarte JL, Faria FA, Ceolin DS, Cestari TM, Assis GF. Effects of passive smoke inhalation on the vocal cords of rats. Rev Bras Otorrinolaringol. 2006;72(2):210-6. http://dx.doi.org/10.1590/S0034-72992006000200010
15. Vasconcelos TB, Andrade KR, Castro CF, Bastos VP. Evaluation of mucociliary transport in trachea of guinea pigs chemically thyroidectomized challenged and sensitized to ovoalbumin [Article in Portuguese]. Rev Biocienc. 2015;21(1):109-17.
16. Bastos VP, Gomes AS, Lima FJ, Brito TS, Soares PM, Pinho JP, et al. Inhaled 1,8-cineole reduces inflammatory parameters in airways of ovalbumin-challenged Guinea pigs. Basic Clin Pharmacol Toxicol. 2011;108(1):34-9. http://dx.doi.org/10.1111/j.1742-7843.2010.00622.x
17. Green LC, Wagner DA, Glogowski J, Skipper PL, Wishnok JS, Tannenbaum SR. Analysis of nitrate, nitrite, and [15N]nitrate in biological fluids. Anal Biochem. 1982;126(1):131-8. http://dx.doi.org/10.1016/0003-2697(82)90118-X
18. Kimoto A, Saitou M, Hirano Y, Iwai T, Tomioka K, Miyata K, et al. A new, simple method for measuring mucociliary clearance in guinea-pigs. Pulm Pharmacol Ther. 1999;12(1):49-54. http://dx.doi.org/10.1006/pupt.1999.0169
19. Bastos VP. Ação broncodilatadora e anti-inflamatória do 1,8 cineol em modelo experimental de asma em cobaias [thesis]. Fortaleza: Universidade Federal do Ceará; 2009.
20. Vasconcelos TB, Ribeiro-Filho HV, Lucetti LT, Magalhães PJ. β-Citronellol, an alcoholic monoterpene with inhibitory properties on the contractility of rat trachea. Braz J Med Biol Res. 2016;49(2):e4800. http://dx.doi.org/10.1590/1414-431x20154800
21. Valença SS, Porto LC. Immunohistochemical study of lung remodeling in mice exposed to cigarette smoke. J Bras Pneumol. 2008;34(10):787-95. http://dx.doi.org/10.1590/S1806-37132008001000006
22. Iwasaki S, Yamakage M, Satoh J, Namiki A. Different inhibitory effects of sevoflurane on hyperreactive airway smooth muscle contractility in ovalbumin-sensitized and chronic cigarette-smoking guinea pig models. Anesthesiology. 2006;105(4):753-63. http://dx.doi.org/10.1097/00000542-200610000-00022
23. Hernandez A, Daffonchio L, Brandolini L, Zuccari G. Effect of a mucoactive compound (CO 1408) on airway hyperreactivity and inflammation induced by passive cigarette smoke exposure in guinea-pigs. Eur Respir J. 1994;7(4):693-7. http://dx.doi.org/10.1183/09031936.94.07040693
24. Campos ML, Corrêa MG, Júnior FH, Casati MZ, Sallum EA, Sallum AW. Cigarette smoke inhalation increases the alveolar bone loss caused by primary occlusal trauma in a rat model. J Periodontal Res. 2014;49(2):179-85. http://dx.doi.org/10.1111/jre.12091
25. Cuzić S, Bosnar M, Kramarić MD, Ferencić Z, Marković D, Glojnarić I, et al. Claudin-3 and Clara cell 10 kDa protein as early signals of cigarette smoke-induced epithelial injury along alveolar ducts. Toxicol Pathol. 2012;40(8):1169-87. http://dx.doi.org/10.1177/0192623312448937
26. Carlos SP, Dias AS, Forgiarini Júnior LA, Patricio PD, Graciano T, Nesi RT, et al. Oxidative damage induced by cigarette smoke exposure in mice: impact on lung tissue and diaphragm muscle. J Bras Pneumol. 2014;40(4):411-20. http://dx.doi.org/10.1590/S1806-37132014000400009
27. Kozma Rde H, Alves EM, Barbosa-de-Oliveira VA, Lopes FD, Guardia RC, Buzo HV, et al. A new experimental model of cigarette smoke-induced emphysema in Wistar rats. J Bras Pneumol. 2014;40(1):46-54. http://dx.doi.org/10.1590/S1806-37132014000100007
28. Banerjee S, Maity P, Mukherjee S, Sil AK, Panda K, Chattopadhyay D, et al. Black tea prevents cigarette smoke-induced apoptosis and lung damage. J Inflamm (Lond). 2007;4:3. http://dx.doi.org/10.1186/1476-9255-4-3
29. Page C, Curtis M, Sutter M, Walker M, Hoffman B. Farmacologia Integrada. 2nd ed. São Paulo: Manole; 2004.
30. Zhong S, Nie YC, Gan ZY, Liu XD, Fang ZF, Zhong BN, et al. Effects of Schisandra chinensis extracts on cough and pulmonary inflammation in a cough hypersensitivity guinea pig model induced by cigarette smoke exposure. J Ethnopharmacol. 2015;165:73-82. http://dx.doi.org/10.1016/j.jep.2015.02.009
31. Ferreira AL, Matsubara LS. Free radicals: concepts, associated diseases, defense system and oxidative stress [Article in Portuguese]. Rev Assoc Med Bras (1992). 1997;43(1):61-8.
32. Vasconcelos TB, Cardoso AR, Josino JB, Macena RH, Bastos VP. Radicais Livres e Antioxidantes: Proteção ou Perigo? UNOPAR Cient Cienc Biol Saude. 2014;16(3):213-9.
33. MacNee W, Rahman I. Is oxidative stress central to the pathogenesis of chronic obstructive pulmonary disease? Trends Mol Med. 2001;7(2):55-62. http://dx.doi.org/10.1016/S1471-4914(01)01912-8
34. MacNee W. Oxidants/antioxidants and COPD. Chest. 2000;117(5 Suppl 1):303S-17S. http://dx.doi.org/10.1378/chest.117.5_suppl_1.303S-a
35. Barreiro E, Peinado VI, Galdiz JB, Ferrer E, Marin-Corral J, Sánchez F, et al. Cigarette smoke-induced oxidative stress: A role in chronic obstructive pulmonary disease skeletal muscle dysfunction. Am J Respir Crit Care Med. 2010;182(4):477-88. http://dx.doi.org/10.1164/rccm.200908-1220OC
36. Camargo Filho JC, Garcia BC, Kodama FY, Bonfim MR, Vanderlei LC, Ramos EM, et al. Effects of aerobic exercise on the skeletal muscle of rats exposed to cigarette smoke. Rev Bras Med Esporte. 2011;17(6):416-9. http://dx.doi.org/10.1590/S1517-86922011000600010
37. Bastos VP, Brito TS, Lima FJ, Pinho JP, Lahlou S, Abreu Matos FJ, et al. Inhibitory effect of 1,8-cineole on guinea-pig airway challenged with ovalbumin involves a preferential action on electromechanical coupling. Clin Exp Pharmacol Physiol. 2009;36(11):1120-6. http://dx.doi.org/10.1111/j.1440-1681.2009.05189.x
38. Somlyo AV, Somlyo AP. Electromechanical and pharmacomechanical coupling in vascular smooth muscle. J Pharmacol Exp Ther. 1968;159(1):129-45.
39. Webb RC. Smooth muscle contraction and relaxation. Adv Physiol Educ. 2003;27(1-4):201-6.
40. James AL, Pare PD, Hogg JC. Effects of lung volume, bronchoconstriction, and cigarette smoke on morphometric airway dimensions. J Appl Physiol (1985). 1988;64(3):913-9.
41. OMINI C, HERNANDEZ A, ZUCCARI G, CLAVENNA G, DAFFONCHIO L. PASSIVE CIGARETTE SMOKE EXPOSURE INDUCES AIRWAY HYPERREACTIVITY TO HISTAMINE BUT NOT TO ACETYLCHOLINE IN GUINEA-PIGS. PULM PHARMACOL. 1990;3(3):145-50. HTTP://DX.DOI.ORG/10.1016/0952-0600(90)90045-K