ABSTRACT
O prejuízo funcional parece diferir entre membros superiores e membros inferiores de pacientes com DPOC. Dois possíveis mecanismos explicam os sintomas importantes de dispneia e fadiga relatados pelos pacientes ao executar tarefas com membros superiores não sustentados: a disfunção neuromecânica dos músculos respiratórios e a alteração dos volumes pulmonares durante as atividades realizadas com membros superiores. A disfunção neuromecânica está relacionada à alteração do padrão respiratório e à simultaneidade de estímulos aferentes e eferentes musculares, o que causaria a dissincronia na ação dos músculos respiratórios em pacientes com DPOC durante esse tipo de exercício. Adicionalmente, o aumento da ventilação durante os exercícios com membros superiores em pacientes com DPOC induz à hiperinsuflação dinâmica em diferentes cargas de trabalho. Nos membros inferiores, há redução da força e da endurance muscular do quadríceps femoral nos pacientes com DPOC comparados a indivíduos saudáveis. Uma explicação para essas reduções é a anormalidade no metabolismo muscular (diminuição da capacidade aeróbia), a dependência do metabolismo glicolítico e o acúmulo rápido de lactato durante o exercício. Quando contrastadas as atividades de membros superiores e membros inferiores, os exercícios com membros superiores resultam em maior demanda metabólica e ventilatória com mais intensa sensação de dispneia e fadiga. Devido às diferenças nas adaptações morfofuncionais dos músculos dos membros superiores e membros inferiores em pacientes com DPOC, protocolos específicos de treinamento de força e/ou endurance devem ser desenvolvidos e testados para os grupos musculares desses segmentos corporais.
Keywords:
Pulmonary disease, chronic obstructive; Muscle fatigue; Upper extremity; Lower extremity.
RESUMO
O prejuízo funcional parece diferir entre membros superiores e membros inferiores de pacientes com DPOC. Dois possíveis mecanismos explicam os sintomas importantes de dispneia e fadiga relatados pelos pacientes ao executar tarefas com membros superiores não sustentados: a disfunção neuromecânica dos músculos respiratórios e a alteração dos volumes pulmonares durante as atividades realizadas com membros superiores. A disfunção neuromecânica está relacionada à alteração do padrão respiratório e à simultaneidade de estímulos aferentes e eferentes musculares, o que causaria a dissincronia na ação dos músculos respiratórios em pacientes com DPOC durante esse tipo de exercício. Adicionalmente, o aumento da ventilação durante os exercícios com membros superiores em pacientes com DPOC induz à hiperinsuflação dinâmica em diferentes cargas de trabalho. Nos membros inferiores, há redução da força e da endurance muscular do quadríceps femoral nos pacientes com DPOC comparados a indivíduos saudáveis. Uma explicação para essas reduções é a anormalidade no metabolismo muscular (diminuição da capacidade aeróbia), a dependência do metabolismo glicolítico e o acúmulo rápido de lactato durante o exercício. Quando contrastadas as atividades de membros superiores e membros inferiores, os exercícios com membros superiores resultam em maior demanda metabólica e ventilatória com mais intensa sensação de dispneia e fadiga. Devido às diferenças nas adaptações morfofuncionais dos músculos dos membros superiores e membros inferiores em pacientes com DPOC, protocolos específicos de treinamento de força e/ou endurance devem ser desenvolvidos e testados para os grupos musculares desses segmentos corporais.
Palavras-chave:
Doença pulmonar obstrutiva crônica; Fadiga muscular; Extremidade superior; Extremidade inferior.
IntroductionProgressive and irreversible evolution is a hallmark of COPD.(1) Because of the primary site of COPD, reduced functional capacity has for many decades been attributed to changes in the respiratory mechanics and impaired pulmonary gas exchange, resulting in dyspnea being the principal symptom limiting exercise. However, in the mid-1990s, one group of authors(2) noted that lower limb fatigue in isolation was greater than was the sensation of dyspnea in 43% of patients with COPD submitted to maximal exercise testing.
Although lower limb muscles are largely responsible for limiting activities such as walking and going up inclines or flights of stairs, it is recognized that the activities of daily living (ADL) that involve the upper limbs, especially those that involve unsupported upper limbs (upper limbs at shoulder level without support), are also poorly tolerated by patients with COPD.(3)
In patients with COPD, the degree of functional impairment appears to differ between the upper and lower limbs and can be explained by the compartment theory. In brief, respiratory muscle function and lower limb muscle function are impaired by structural and functional changes. Upper limb muscle structure and function are relatively preserved, which is due to the maintenance of ADLs that involve the arms or to the use of some of those muscles during the work of breathing.(4)
Structural changes in the lower limb muscles result in reduced overall exercise capacity, progressing to deconditioning caused by a reduction in the performance of physical ADLs in order to avoid dyspnea.(5) The performance of physical ADLs appears to be a determining factor in muscle changes.(6) However, such changes are also thought to be modulated by other local and systemic factors, such as inflammation, oxidative stress, pharmacological treatment, and nutritional disorders.(6)
Although various researchers have studied the effects that lower limb and upper limb exercises have on various physiological and clinical outcomes,(7-16) only a few studies have drawn comparisons between lower limb muscle function and upper limb muscle function.(17,18) Only one study compared lower and upper limb exercises in terms of metabolic and ventilatory demands, as well as in terms of perceived effort.(18)
Impact of upper limb activities on metabolic and ventilatory responsesAlthough upper limb mechanical efficiency and exercise capacity are relatively preserved in patients with COPD,(19) these patients complain of severe dyspnea while performing, without upper limb support, tasks that could be considered trivial. There are two mechanisms to which that finding might be attributable: neuromechanical dysfunction (thoracoabdominal asynchrony) of respiratory muscles (diaphragm and accessory muscles)(20,21); and changes in lung volume during activities involving the upper limbs.(22-28)
Neuromechanical dysfunction was first described in a study conducted by Tangri Woolf.(21) In that study, the breathing patterns of 12 patients with COPD were evaluated by inductance plethysmography. A rapid, shallow breathing pattern was observed during bending, tying shoelaces, tooth brushing, and hair combing. Fluoroscopy revealed an irregular pattern of diaphragm movement during hair brushing and tooth brushing in the orthostatic position. That was followed by a period during which ventilation increased. The authors stated that this might be due to carbon dioxide retention and decreased arterial oxygen pressure during those activities. Therefore, the hyperventilation observed after the activity had been discontinued would have been required in order to allow blood gases to return to baseline levels. The dyspnea observed in COPD patients after activities involving the upper limbs might be due to increased RR associated with low tidal volume.
One group of authors found a rapid, shallow breathing pattern in 34 COPD patients (FEV1, 0.95 ± 0.08 L) who kept their upper limbs raised to shoulder height, with the elbows held at a 90° angle, for two minutes,(28) thereby corroborating the findings of Tangri Woolf.(21) The authors attributed the change in the breathing pattern to the simultaneity of afferent and efferent muscle stimuli, which could cause respiratory muscle asynchrony during unsupported upper limb exercise in patients with COPD.
According to Breslin,(29) the thoracoabdominal asynchrony triggered by unsupported upper limb exercises causes dyspnea in patients with COPD. The author found that patients with severe COPD (FEV1, 37 ± 3% of predicted) reported a marked increase in the sensation of dyspnea during routine tasks involving the upper limbs, especially during activities that required unsupported elevation of the upper limbs. The author speculated that dyspnea is associated with changes in respiratory muscle function, i.e., decreased respiratory muscle strength and endurance, as well as with an increase in the recruitment of rib cage muscles and accessory muscles during those activities. Unsupported upper limb exercise further impairs the ability of respiratory muscles to aid in ventilation because this type of exercise requires the recruitment of respiratory muscles concomitantly with the maintenance of chest wall stability.
In another study,(30) healthy individuals (n = 18) were compared with COPD patients (n = 25; FEV1, 38 ± 5% of predicted) in terms of their performance on an unsupported activity involving the arm flexor muscles during the expiratory phase. Muscle activity was evaluated by electromyography (EMG), the pattern of thoracoabdominal motion was measured by inductance plethysmography, and the sensation of dyspnea was evaluated by a visual analog scale. Upper limb muscle endurance during unsupported exercises was reported to be reduced in healthy individuals and in patients with COPD, being associated with increased dyspnea and changes in the pattern of respiratory muscle recruitment. Unsupported exercise resulted in a significant increase in the mean EMG signal amplitude of the diaphragm during inhalation and exhalation, as well as in thoracoabdominal motion asynchrony and increased intensity of dyspnea. These changes in diaphragmatic recruitment and thoracoabdominal motion can partially explain the increase in the intensity of dyspnea caused by unsupported upper limb exercise.
The effects of upper limb flexion on metabolic responses-oxygen consumption (VO2) and carbon dioxide production (VCO2)-and ventilatory responses (minute ventilation, tidal volume, and RR) have also been evaluated in a study of patients with COPD.(24) Those variables were studied while the patients held their upper limbs in three different positions, in sequence: neutral (upper limbs resting along the body); horizontal flexion at shoulder level for two minutes; and then neutral again. The principal finding of that study was that unsupported upper limb exercise increased minute ventilation by increasing the RR, as evidenced by the activity of the sternocleidomastoid muscle, resulting in a minimal increase in tidal volume.
Higher metabolic and ventilatory demands were also reported in a study(25) comparing patients with COPD (FEV1, 38 ± 13% of predicted) and healthy elderly individuals. The authors found a significant increase in baseline VO2, VCO2, pulmonary ventilation, RR, and HR in COPD patients during three basic upper limb elevations (shoulder flexion at 90°, shoulder flexion at 180°, and shoulder abduction at 90°). The increase explained the higher metabolic and respiratory demands.
According to one group of authors,(26) unsupported upper limb exercise alters respiratory muscle recruitment and causes dyspnea in patients with COPD. The authors evaluated 11 COPD patients (FEV1, 0.65 ± 0.21 L) at rest, during unsupported upper limb exercise, and during supported exercise on an arm ergometer. The duration of the exercise, HR, VO2, and pulmonary ventilation at peak exertion were lower during unsupported upper limb endurance exercise than during supported upper limb exercise. In 8 of the patients, gastric pressure increased at the end of inhalation, and pleural pressure was less negative during the unsupported exercise. Those pressure changes were attributed to the ventilatory overload of the inspiratory muscles of the rib cage and diaphragm. The change in the recruitment of respiratory muscles could explain why certain unsupported upper limb exercises are poorly tolerated by patients with COPD.
In agreement with the previous findings, another group of authors(23) also suggested that the limitation on unsupported upper limb exercise in COPD patients is characterized by thoracoabdominal asynchrony of inspiratory muscles. Those authors studied the relationship between pulmonary function at rest and unsupported upper limb exercise performance (upper limb flexion at shoulder level) in patients with COPD (n = 21; FEV1, 42 ± 12% of predicted). The duration of upper limb exercise significantly correlated with inspiratory capacity (IC), functional residual capacity (FRC), maximum inspiratory pressure, and FEV1. In addition, arm circumference and VO2 correlated with the duration of the exercise. The variables IC, FRC, and FEV1 collectively accounted for 77% of the variation in exercise duration. In that context, the authors reported that therapeutic strategies aimed at increasing IC, decreasing FRC, or increasing inspiratory muscle strength and upper limb strength could provide symptom relief and improve unsupported upper limb exercise performance in patients with
COPD.
According to one group of authors,(14) arm ergometer incremental exercise resulted in dynamic hyperinflation in patients with moderate to severe obstruction. The hyperinflation correlated with an increase in dyspnea, upper limb effort, and VO2 at peak exertion. Increased ventilation during upper limb exercises in patients with COPD has been associated with dynamic hyperinflation and decreased IC at different workloads.(12)
The abovementioned studies analyzed the upper limbs while they were held motionless or during cycle ergometer exercises, neither of which is representative of ADLs involving the upper limbs. In this context, Velloso et al.(31) were the first to study metabolic and ventilatory outcomes during the performance of ADLs, such as sweeping the floor, erasing a blackboard, lifting jars with different loads, and changing light bulbs, in patients with COPD (FEV1, 32.5 ± 1.8% of predicted). Maximal VO2 and pulmonary ventilation were significantly greater during those activities than during activities performed at rest. In addition, when compared with the control group, patients with moderate to severe COPD reported a higher rate of fatigue and greater sensation of dyspnea during the ADLs that required upper limb elevation.
There have been only a few studies analyzing the microstructure of the upper limb or shoulder girdle muscles. There seems to be no change in the relative proportion of fibers in the biceps brachii; however, type I and type II atrophic fibers are evident, especially in patients with weight loss and airflow obstruction that is more severe.(32)
It appears that oxidative capacity is preserved or even increased in the deltoid muscles of patients with COPD,(33) as opposed to what is observed in lower limb muscles (Figure 1). One possible explanations for this difference is that patients continue to perform, to a certain extent, the ADLs that involve the upper limbs but reduce their performance of the activities that involve the lower limbs, adopting a sedentary lifestyle in order to minimize dyspnea. In a more detailed study, the analysis of biopsy samples from the middle deltoid muscle of the non-dominant limb revealed three different types of fibers in COPD patients (fibers of normal diameter, atrophic fibers, and hypertrophic fibers), resulting in a mosaic pattern.(34) That finding was prevalent in patients with severe COPD.
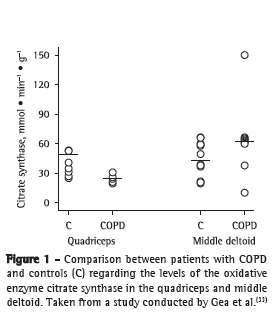
Unlike the lower limbs, the upper limbs have not been studied in terms of the objective assessment of muscle fatigue. However, there have been numerous studies analyzing the ventilatory and metabolic responses during upper limb exercise in patients with COPD. It has been reported that VO2, VCO2, and pulmonary ventilation at peak exertion are lower during upper limb exercise than during lower limb exercise.(35) However, at comparable workloads (similar VO2), upper limb exercise leads to greater VCO2, ventilation, and lactate production than does lower limb exercise,(36) with an early anaerobic threshold. This is probably due to reduced muscle mass and possible microstructural changes, which result in increased muscle work in order to maintain the exercise. Therefore, the activities that involve shoulder girdle muscles lead to a marked sensation of dyspnea, as well as to upper limb fatigue.
Lower limb muscle dysfunction in patients with COPDBecause the quadriceps is readily accessible and a primary muscle of locomotion, it is the muscle that is most commonly studied in COPD patients with skeletal muscle dysfunction.(37)
Gosselink et al.(38) were the first to document reduced quadriceps muscle strength, as assessed by isometric peak torque (PT), in patients with COPD, showing it to be a predictor of reduced maximal VO2. In addition, patients present with decreased muscle endurance when compared with healthy individuals. A plausible explanation for this reduction is abnormal muscle metabolism in which the oxidative activity is lower.(39) It is of note that there is an association between muscle endurance and the level of physical ADL performance, as well as between muscle endurance and the degree of airflow obstruction.(40) Certain factors, such as reduced muscle strength, decreased aerobic capacity, dependence on glycolytic metabolism, and rapid accumulation of lactate during exercise, might be responsible for early muscle fatigue in patients with COPD.
Muscle fatigue has been evaluated by surface EMG (sEMG) associated with voluntary contraction measurements. More recently, muscle fatigue has been evaluated by magnetic stimulation of the femoral nerve, a technique that is considered the gold standard because it is non-volitional. Muscle contraction in response to the magnetic stimulus is known as twitch tension. A study comparing patients with COPD and healthy elderly individuals in terms of quadriceps muscle strength, as evaluated by magnetic stimulation and sEMG, showed a 30% reduction, on average, in quadriceps muscle strength in the patients with COPD; the reduction was attributed to a decrease in the proportion of type I fibers and an increase in the proportion of type II fibers, as well as to reduced oxidative metabolism and quadriceps muscle deconditioning.(37) Similar results were found by a group of authors(41) investigating reduced lower limb muscle endurance in 36 patients with COPD (FEV1, 36 ± 14% of predicted) and in 39 healthy individuals. Quadriceps strength and endurance were both evaluated. In the patients with COPD, quadriceps strength was reduced to 43% after exercise, whereas it remained at 77% in the control patients. The reduction in muscle endurance was independent of the pulmonary function of the patient or the force generated by the quadriceps.
One group of authors(42) compared patients with COPD (FEV1, 36 ± 5% of predicted) and 9 healthy individuals in terms of the degree of fatigue caused by cycle ergometer exercise. Fatigue was evaluated by magnetic stimulation of the femoral nerve after cycle ergometer exercise at 60% of the maximum workload. Fatigue, as assessed by a drop in twitch tension, was greater in the patients with COPD than in the healthy individuals. A drop in quadriceps twitch tension was also reported in another study.(43) In that study, quadriceps muscle fatigue was evaluated with magnetic stimulation of the femoral nerve before and after submaximal cycle ergometer exercise in 30 patients with COPD (FEV1, 42 ± 3% of predicted). The authors found that the decline in quadriceps muscle strength was associated with a drop in the median EMG signal frequency, with an increase in the sensation of lower limb fatigue, together with an increase in arterial blood lactate levels.
In one study, patients with COPD were reported to present with quadriceps muscle atrophy, as shown by a CT scan of the thigh (66 cm2 vs. 107 cm2 in healthy individuals). The authors found that, in the patients with COPD, quadriceps muscle strength was reduced by 17 kg on average, and the endurance test showed that the time to fatigue was 38 s shorter in the patients with COPD than in healthy individuals.(44)
A study comparing changes in the six-minute walk test performance with those in the shuttle walk test performance in 14 patients with COPD (FEV1, 50 ± 8% of predicted) provided an analysis of the response to dyspnea, as well as of the level of quadriceps muscle fatigue. The authors used sEMG and magnetic stimulation of the femoral nerve, contractile muscle fatigue being defined as a 15% reduction in post-exercise strength when compared with that measured at rest. We find it curious that post-exercise dyspnea was similar between the two tests, that the test did not cause a significant drop in quadriceps twitch tension, and that muscle fatigue occurred in less than 15% of the patients after the two walk tests.(45)
The principal changes in the quadriceps muscle and middle deltoid muscle are shown in Table 1. Such changes increase quadriceps muscle fatigability, as demonstrated during cycle ergometer exercise at a constant workload(43,55,56) and during localized exercise.(57)
Comparison between upper limb and lower limb exercise response in COPDIt is of note that, although patients with COPD have been reported to present with impaired lower limb and shoulder girdle muscles, the morphological adaptations appear to differ between these muscles (Table 1).
Celli et al.(22) were the first to compare lower limb and upper limb activities in patients with COPD. The authors noted that, despite the lower amount of work, the time during which patients were able to maintain the upper limbs raised without support during the activity of changing rings on a vertical plank was lower than was that during which patients were able to perform lower limb exercise on a cycle ergometer. Unsupported upper limb activities in patients with COPD end before lower limb exercises do, although the HR and VO2 obtained during upper limb exercise are lower. It appears that the limiting factor for upper limb exercise in some patients is the early onset of intense dyspnea, which is associated with thoracoabdominal asynchrony.
One group of authors(18) evaluated peripheral muscle weakness and endurance of the upper and lower limbs (biceps brachii and quadriceps) in patients with COPD. A drop in PT over the course of 14 isokinetic contractions was used as a criterion for fatigue. Biceps brachii fatigue was found to be greater than was quadriceps fatigue, the drop in PT being 42% and 28%, respectively.
The authors of one study(57) determined the degree of respiratory and peripheral muscle weakness in patients with moderate to severe COPD. The study compared 22 healthy elderly individuals and 40 patients with COPD (FEV1, 41 ± 19% of predicted) in terms of respiratory and peripheral muscle strength. The authors assessed pulmonary function, isometric strength of four muscle groups (hand-grip strength, elbow flexion, shoulder abduction, and knee extension), neck flexion strength, maximal inspiratory pressure, and maximal expiratory pressure. As expected, respiratory and peripheral muscle strength was lower in the patients with COPD patients than in the normal individuals. In patients with stable COPD, muscle weakness did not affect all muscles similarly. Inspiratory muscle strength was more affected than was peripheral muscle strength, whereas proximal upper limb muscle strength was more affected than was distal upper limb muscle strength. Although those findings were not discussed by the authors, reduced respiratory muscle strength in COPD patients has been reported to be due to lung hyperinflation, which leads to mechanical impairment.(58) Distal strength is possibly preserved because patients are able to continue to perform ADLs that involve repeated hand-grip movements, including gripping, holding, and carrying objects. In contrast, reduced proximal upper limb muscle strength, as assessed by shoulder abduction, can be explained by a reduction in unsupported arm activities in order to avoid dyspnea. More recently, for the same relative load, dyspnea scores and lactate production were found to be higher during upper limb exercise performed on an arm ergometer than during lower limb exercise performed on a cycle ergometer.(59) One study reported that lung hyperinflation, as assessed by reduced IC, was greater after upper limb exercise than after a treadmill walk test, the two tests having been performed at similar metabolic loads.(21) The comparison between reduced upper limb muscle strength (elbow flexors) and reduced lower limb muscle strength (knee extensors) after a fatigue protocol in 8 patients with moderate to severe COPD showed an increase in perception of fatigue scale scores (for elbow flexors and knee extensors) before and after the protocol.(60) In the patients with COPD, the fatigability of the elbow flexors was greater than was that of the knee extensors.
Final considerationsUpper limb strength and endurance are lower in COPD patients than in healthy individuals, as are lower limb strength and endurance. Regarding the upper limbs, two possible mechanisms are related to those findings: neuromechanical dysfunction (thoracoabdominal asynchrony) of respiratory muscles (diaphragm and accessory muscles); and changes in lung volume during activities involving the upper limbs. Regarding the lower limbs, reduced muscle strength and endurance are related to decreased muscle mass, decreased aerobic capacity, a predominance of glycolytic metabolism, and rapid accumulation of lactate during exercise, factors that might be responsible for early muscle fatigue in COPD patients.
Because of the continuing controversy over the distribution of muscle dysfunction between the upper and lower limbs in patients with COPD, there is a need for further studies, comparing macrostructural and microstructural changes while adjusting for certain factors, such as disease severity and phenotype, as well as the presence or absence of hypoxemia and muscle depletion.
References1. Fabbri LM, Hurd SS; GOLD Scientific Committee. Global Strategy for the Diagnosis, Management and Prevention of COPD: 2003 update. Eur Respir J. 2003;22(1):1-2.
2. Hamilton AL, Killian KJ, Summers E, Jones NL. Symptom intensity and subjective limitation to exercise in patients with cardiorespiratory disorders. Chest. 1996;110(5):1255-63.
3. Dolmage TE, Maestro L, Avendano MA, Goldstein RS. The ventilatory response to arm elevation of patients with chronic obstructive pulmonary disease. Chest. 1993;104(4):1097-100.
4. Gea J, Orozco-Levi M, Barreiro E, Ferrer A, Broquetas J. Structural and functional changes in the skeletal muscles of COPD patients: the "compartments" theory. Monaldi Arch Chest Dis. 2001;56(3):214-24.
5. Gosker HR, Schols AM. Fatigued muscles in COPD but no finishing line in sight. Eur Respir J. 2008;31(4):693-4.
6. Doucet M, Dubé A, Joanisse DR, Debigaré R, Michaud A, Paré MÈ, et al. Atrophy and hypertrophy signalling of the quadriceps and diaphragm in COPD. Thorax. 2010;65(11):963-70.
7. Vieira L, Bottaro M, Celes R, Viegas CA, e Silva CA. Isokinetic muscle evaluation of quadriceps in patients with chronic obstructive pulmonary disease. Rev Port Pneumol. 2010;16(5):717-36.
8. Mercken EM, Gosker HR, Rutten EP, Wouters EF, Bast A, Hageman GJ, et al. Systemic and pulmonary oxidative stress after single-leg exercise in COPD. Chest. 2009;136(5):1291-300.
9. Gagnon P, Saey D, Vivodtzev I, Laviolette L, Mainguy V, Milot J, et al. Impact of preinduced quadriceps fatigue on exercise response in chronic obstructive pulmonary disease and healthy subjects. J Appl Physiol. 2009;107(3):832-40.
10. Zakynthinos SG, Vogiatzis I. The major limitation to exercise performance in COPD is inadequate energy supply to the respiratory and locomotor muscles vs. lower limb muscle dysfunction vs. dynamic hyperinflation. Exercise intolerance in COPD: putting the pieces of the puzzle together. J Appl Physiol. 2008;105(2):760.
11. Gosker HR, Hesselink MK, Duimel H, Ward KA, Schols AM. Reduced mitochondrial density in the vastus lateralis muscle of patients with COPD. Eur Respir J. 2007;30(1):73-9.Erratum in: Eur Respir J. 2007;30(4):817.
12. Colucci M, Cortopassi F, Porto E, Castro A, Colucci E, Iamonti VC, et al. Upper limb exercises using varied workloads and their association with dynamic hyperinflation in patients with COPD. Chest. 2010;138(1):39-46.
13. O'Shea SD, Taylor NF, Paratz JD. Measuring muscle strength for people with chronic obstructive pulmonary disease: retest reliability of hand-held dynamometry. Arch Phys Med Rehabil. 2007;88(1):32-6.
14. Vitacca M, Bianchi L, Sarvà M, Paneroni M, Balbi B. Physiological responses to arm exercise in difficult to wean patients with chronic obstructive pulmonary disease. Intensive Care Med. 2006;32(8):1159-66.
15. Zhan S, Cerny FJ, Gibbons WJ, Mador MJ, Wu YW. Development of an unsupported arm exercise test in patients with chronic obstructive pulmonary disease. J Cardiopulm Rehabil. 2006;26(3):180-7; discussion 188-90.
16. Gigliotti F, Coli C, Bianchi R, Grazzini M, Stendardi L, Castellani C, et al. Arm exercise and hyperinflation in patients with COPD: effect of arm training. Chest. 2005;128(3):1225-32.
17. Yoshikawa M, Yoneda T, Takenaka H, Fukuoka A, Okamoto Y, Narita N, et al. Distribution of muscle mass and maximal exercise performance in patients with COPD. Chest. 2001;119(1):93-8.
18. Gosker HR, Lencer NH, Franssen FM, van der Vusse GJ, Wouters EF, Schols AM. Striking similarities in systemic factors contributing to decreased exercise capacity in patients with severe chronic heart failure or COPD. Chest. 2003;123(5):1416-24.
19. Porto EF, Castro AA, Velloso M, Nascimento O, Dal Maso F, Jardim JR. Exercises using the upper limbs hyperinflate COPD patients more than exercises using the lower limbs at the same metabolic demand. Monaldi Arch Chest Dis. 2009;71(1):21-6.
20. Franssen FM, Wouters EF, Baarends EM, Akkermans MA, Schols AM. Arm mechanical efficiency and arm exercise capacity are relatively preserved in chronic obstructive pulmonary disease. Med Sci Sports Exerc. 2002;34(10):1570-6.
21. Tangri S, Woolf CR. The breathing pattern in chronic obstructive lung disease during the performance of some common daily activities. Chest. 1973;63(1):126-7.
22. Celli BR, Rassulo J, Make BJ. Dyssynchronous breathing during arm but not leg exercise in patients with chronic airflow obstruction. N Engl J Med. 1986;314(23):1485-90.
23. Lebzelter J, Klainman E, Yarmolovsky A, Sulkes J, Fink-Krelbaum T, Kramer MR, et al. Relationship between pulmonary function and unsupported arm exercise in patients with COPD. Monaldi Arch Chest Dis. 2001;56(4):309-14.
24. Martinez FJ, Couser JI, Celli BR. Respiratory response to arm elevation in patients with chronic airflow obstruction. Am Rev Respir Dis. 1991;143(3):476-80.
25. Baarends EM, Schols AM, Slebos DJ, Mostert R, Janssen PP, Wouters EF. Metabolic and ventilatory response pattern to arm elevation in patients with COPD and healthy age-matched subjects. Eur Respir J. 1995;8(8):1345-51.
26. Criner GJ, Celli BR. Effect of unsupported arm exercise on ventilatory muscle recruitment in patients with severe chronic airflow obstruction. Am Rev Respir Dis. 1988;138(4):856-61.
27. Gigliotti F, Coli C, Bianchi R, Grazzini M, Stendardi L, Castellani C, et al. Arm exercise and hyperinflation in patients with COPD: effect of arm training. Chest. 2005;128(3):1225-32.
28. Epstein SK, Celli BR, Williams J, Tarpy S, Roa J, Shannon T. Ventilatory response to arm elevation. Its determinants and use in patients with chronic obstructive pulmonary disease. Am J Respir Crit Care Med. 1995;152(1):211-6.
29. Breslin EH. Dyspnea-limited response in chronic obstructive pulmonary disease: reduced unsupported arm activities. Rehabil Nurs. 1992;17(1):12-20.
30. Breslin EH, Garoutte BC. Respiratory responses to unsupported arm lifts paced during expiration. West J Nurs Res. 1995;17(1):91-100; discussion 101-11.
31. Velloso M, Stella SG, Cendon S, Silva AC, Jardim JR. Metabolic and ventilatory parameters of four activities of daily living accomplished with arms in COPD patients. Chest. 2003;123(4):1047-53.
32. Sato Y, Asoh T, Honda Y, Fujimatsu Y, Higuchi I, Oizumi K. Morphologic and histochemical evaluation of muscle in patients with chronic pulmonary emphysema manifesting generalized emaciation. Eur Neurol. 1997;37(2):116-21.
33. Gea JG, Pasto M, Carmona MA, Orozco-Levi M, Palomeque J, Broquetas J. Metabolic characteristics of the deltoid muscle in patients with chronic obstructive pulmonary disease. Eur Respir J. 2001;17(5):939-45.
34. Hernández N, Orozco-Levi M, Belalcázar V, Pastó M, Minguella J, Broquetas JM, et al. Dual morphometrical changes of the deltoid muscle in patients with COPD. Respir Physiol Neurobiol. 2003;134(3):219-29.
35. Celli BR. The clinical use of upper extremity exercise. Clin Chest Med. 1994;15(2):339-49.
36. Davis JA, Vodak P, Wilmore JH, Vodak J, Kurtz P. Anaerobic threshold and maximal aerobic power for three modes of exercise. J Appl Physiol. 1976;41(4):544-50.
37. Man WD, Soliman MG, Nikoletou D, Harris ML, Rafferty GF, Mustfa N, et al. Non-volitional assessment of skeletal muscle strength in patients with chronic obstructive pulmonary disease. Thorax. 2003;58(8):665-9.
38. Gosselink R, Troosters T, Decramer M. Peripheral muscle weakness contributes to exercise limitation in COPD. Am J Respir Crit Care Med. 1996;153(3):976-80.
39. Serres I, Gautier V, Varray A, Préfaut C. Impaired skeletal
muscle endurance related to physical inactivity and altered lung function in COPD patients. Chest. 1998;113(4):900-5.
40. Janaudis-Ferreira T, Wadell K, Sundelin G, Lindström B. Thigh muscle strength and endurance in patients with COPD compared with healthy controls. Respir Med. 2006;100(8):1451-7.
41. Coronell C, Orozco-Levi M, Méndez R, Ramírez-Sarmiento A, Gáldiz JB, Gea J. Relevance of assessing quadriceps endurance in patients with COPD. Eur Respir J. 2004;24(1):129-36.
42. Mador MJ, Bozkanat E, Kufel TJ. Quadriceps fatigue after cycle exercise in patients with COPD compared with healthy control subjects. Chest. 2003;123(4):1104-11.
43. Saey D, Côté CH, Mador MJ, Laviolette L, LeBlanc P, Jobin J, et al. Assessment of muscle fatigue during exercise in chronic obstructive pulmonary disease. Muscle Nerve. 2006;34(1):62-71.
44. Allaire J, Maltais F, Doyon JF, Noël M, LeBlanc P, Carrier G, et al. Peripheral muscle endurance and the oxidative profile of the quadriceps in patients with COPD. Thorax. 2004;59(8):673-8.
45. Pepin V, Brodeur J, Lacasse Y, Milot J, Leblanc P, Whittom F, et al. Six-minute walking versus shuttle walking: responsiveness to bronchodilation in chronic obstructive pulmonary disease. Thorax. 2007;62(4):291-8.
46. Schols AM, Wouters EF, Soeters PB, Westerterp KR. Body composition by bioelectrical-impedance analysis compared with deuterium dilution and skinfold anthropometry in patients with chronic obstructive pulmonary disease. Am J Clin Nutr. 1991;53(2):421-4.
47. Clark CJ, Cochrane LM, Mackay E, Paton B. Skeletal muscle strength and endurance in patients with mild COPD and the effects of weight training. Eur Respir J. 2000;15(1):92-7.
48. Van't Hul A, Harlaar J, Gosselink R, Hollander P, Postmus P, Kwakkel G. Quadriceps muscle endurance in patients with chronic obstructive pulmonary disease. Muscle Nerve. 2004;29(2):267-74.
49. Maltais F, Simard AA, Simard C, Jobin J, Desgagnés P, LeBlanc P. Oxidative capacity of the skeletal muscle and lactic acid kinetics during exercise in normal subjects and in patients with COPD. Am J Respir Crit Care Med. 1996;153(1):288-93.
50. Jakobsson P, Jorfeldt L, Henriksson J. Metabolic enzyme activity in the quadriceps femoris muscle in patients with severe chronic obstructive pulmonary disease. Am J Respir Crit Care Med. 1995;151(2 Pt 1):374-7.
51. Hildebrand IL, Sylvén C, Esbjörnsson M, Hellström K, Jansson E. Does chronic hypoxaemia induce transformations of fibre types? Acta Physiol Scand. 1991;141(3):435-9.
52. Satta A, Migliori GB, Spanevello A, Neri M, Bottinelli R, Canepari M, et al. Fibre types in skeletal muscles of chronic obstructive pulmonary disease patients related to respiratory function and exercise tolerance. Eur Respir J. 1997;10(12):2853-60.
53. Saey D, Lemire BB, Gagnon P, Bombardier E, Tupling AR, Debigaré R, et al. Quadriceps metabolism during constant workrate cycling exercise in chronic obstructive pulmonary disease. J Appl Physiol. 2011;110(1):116-24.
54. Whittom F, Jobin J, Simard PM, Leblanc P, Simard C, Bernard S, et al. Histochemical and morphological characteristics of the vastus lateralis muscle in patients with chronic obstructive pulmonary disease.
Med Sci Sports Exerc. 1998;30(10):1467-74.
55. Saey D, Debigare R, LeBlanc P, Mador MJ, Cote CH, Jobin J, et al. Contractile leg fatigue after cycle exercise: a factor limiting exercise in patients with chronic obstructive pulmonary disease. Am J Respir Crit Care Med. 2003;168(4):425-30.
56. Sillen MJ, Janssen PP, Akkermans MA, Wouters EF, Spruit MA. The metabolic response during resistance training and neuromuscular electrical stimulation (NMES) in patients with COPD, a pilot study. Respir Med. 2008;102(5):786-9.
57. Gosselink R, Troosters T, Decramer M. Distribution of muscle weakness in patients with stable chronic obstructive pulmonary disease. J Cardiopulm Rehabil. 2000;20(6):353-60.
58. Rochester DF. Tests of respiratory muscle function. Clin Chest Med. 1988;9(2):249-61.
59. Castagna O, Boussuges A, Vallier JM, Prefaut C, Brisswalter J. Is impairment similar between arm and leg cranking exercise in COPD patients? Respir Med. 2007;101(3):547-53.
60. Possani HV, Carvalho MJ, Probst VS, Pitta F, Brunetto AF. Comparação da redução na força muscular de membros superiores e membros inferiores após um protocolo de fadiga em pacientes com Doença Pulmonar Obstrutiva Crônica (DPOC). ASSOBRAFIR Ciência. 2009(1);33-43.
* Study carried out at Nove de Julho University, São Paulo, Brazil.
Correspondence to: Simone Dal Corso. Avenida Francisco Matarazzo, 612, 1º andar, Água Branca, CEP 05001-100, São Paulo, SP, Brasil.
Fax: 55 11 3665-9325. E-mail: simonedc@uninove.br
Financial support: Eduardo Foschini Miranda is the recipient of a scholarship from the Fundação de Amparo à Pesquisa do Estado de São Paulo (FAPESP, Foundation for the Support of Research in the state of São Paulo); Grant no. 2009/03586-0.
Submitted: 29 November 2010. Accepted, after review: 10 March 2011.
About the authorsEduardo Foschini Miranda
Doctoral Student. Doctoral Program in Rehabilitation Sciences, Nove de Julho University, São Paulo, Brazil.
Carla Malaguti
Professor. Master's Program in Rehabilitation Sciences, Nove de Julho University, São Paulo, Brazil.
Simone Dal Corso
Professor. Master's and Doctoral Program in Rehabilitation Sciences, Nove de Julho University, São Paulo, Brazil.