ABSTRACT
Objective: To compare the behavior of the oxygen uptake efficiency slope (OUES) with that of oxygen uptake at peak exertion (VO2peak). Methods: This was a prospective cross-sectional study involving 21 patients (15 men) with mild-to-moderate COPD undergoing spirometry, handgrip strength (HGS) testing, cardiopulmonary exercise testing, and determination of lactate at peak exertion (LACpeak). Results: Mean weight was 66.7 ± 13.6 kg, and mean age was 60.7 ± 7.8 years. With the exception of FEV1 and FEV1/FVC ratio (75.8 ± 18.6% of predicted and 56.6 ± 8.8%, respectively), all spirometric variables were normal, as was HGS. The patients exhibited significant metabolic and hemodynamic stress, as evidenced by the means (% of predicted) for VO2peak (93.1 ± 15.4), maximum HR (92.5 ± 10.4), and OUES (99.4 ± 24.4), as well as for the gas exchange rate (1.2 ± 0.1). The correlation between VO2peak and OUES was significant (r = 0.747; p < 0.0001). The correlation between HGS and VO2peak (r = 0.734; pX< 0.0001) was more significant than was that between HGS and OUES (r = 0.453; p < 0.05). Similar results were found regarding the correlations of VO2peak and OUES with MIP. Although LACpeak correlated significantly with VO2peak (r = −0.731; p < 0.0001), only LACpeak/maximum power correlated significantly with OUES (r = −0.605; p = 0.004). Conclusions: Our findings suggest that, in mild-to-moderate COPD, VO2 determinants other than overall muscle strength have a greater impact on OUES than on VO2peak.
Keywords:
Respiratory function tests; Pulmonary disease, chronic obstructive; Muscle strength; Oxygen consumption.
RESUMO
Objetivo: Comparar o comportamento de oxygen uptake efficiency slope (OUES, inclinação da eficiência do consumo de oxigênio) com o do consumo de oxigênio no pico do exercício (VO2pico). Métodos: Estudo prospectivo transversal envolvendo 21 pacientes (15 homens) com DPOC leve/moderada que foram submetidos a espirometria, dinamometria de preensão palmar (DIN), teste cardiopulmonar de exercício e medida de lactato no pico do exercício (LACpico). Resultados: A média de peso foi 66,7 ± 13,6 kg, e a de idade foi 60,7 ± 7,8 anos. Com exceção de VEF1 e relação VEF1/CVF (75,8 ± 18,6 do previsto e 56,6 ± 8,8, respectivamente), as demais variáveis espirométricas foram normais, assim como DIN. As médias, em % do previsto, para VO2pico (93,1 ± 15,4), FC máxima (92,5 ± 10,4) e OUES (99,4 ± 24,4), assim como a da taxa de troca respiratória (1,2 ± 0,1), indicaram estresse metabólico e hemodinâmico importante. A correlação entre o VO2pico e a OUES foi elevada (r = 0,747; p < 0,0001). A correlação entre DIN e VO2pico (r = 0,734; p < 0,0001) foi mais expressiva do que com aquela entre DIN e OUES (r = 0,453; p < 0,05). Resultados semelhantes ocorreram em relação às correlações de VO2pico e OUES com PImáx. Houve correlação significativa entre VO2pico e LACpico (r = −0,731; p < 0,0001), mas essa só ocorreu entre OUES e LACpico/potência máxima (r = −0,605; p = 0,004). Conclusões: Nossos resultados sugerem que, na DPOC leve/moderada, determinantes do VO2, além da força muscular global, têm um maior impacto na OUES do que no VO2pico.
Palavras-chave:
Testes de função respiratória; Doença pulmonar obstrutiva crônica; Força muscular; Consumo de oxigênio.
IntroductionWorldwide, COPD is one of the leading causes of morbidity and mortality, causing significant musculoskeletal morbidity and reducing quality of life.(1) The main methods for evaluating and monitoring the effectiveness of COPD treatment are assessment of exercise capacity and assessment of respiratory and peripheral muscle strength.(1) Cardiopulmonary exercise testing (CPET) is one of the most important methods in this context because it combines comprehensive cardiopulmonary and metabolic analyses, including oxygen consumption (VO2) and gas exchange; however, useful variables such as oxygen uptake efficiency have been little studied in patients with COPD.
Oxygen uptake efficiency, i.e., the VO2 required in order to meet a given ventilatory demand, can be determined by calculating the ratio between VO2 and minute ventilation (VE) or by log-transforming VE (log10VE), thus obtaining the derivative of the VO2/VE ratio-known as the oxygen uptake efficiency slope (OUES)-as proposed by Baba et al.(2) A more recent study has described another way of determining the relationship between VO2 and VE, i.e., by calculating the oxygen uptake efficiency plateau.(3)
The OUES can play an important role in the assessment of aerobic capacity based on submaximal data obtained after pharmacological or non-pharmacological intervention in patients whose exercise capacity is more limited. Maximal effort on CPET might be inappropriate, increasing risks and patient discomfort. In addition, stage I/II COPD commonly overlaps with other diseases, such as heart failure, diabetes, and peripheral vascular disease, the presence of multiple comorbidities therefore compromising the assessment of maximal exercise capacity.(4)
Although some studies have analyzed the OUES in normal individuals and in adults with heart failure,(5,6) only one study has done so in patients with COPD.(7) The study focused on the role of dynamic hyperinflation (DH) in reducing the OUES, the role of muscle strength not having been evaluated. Considering that the OUES is independent of maximal effort, the authors suggested that DH plays an important role in decreasing cardiac output (indirectly assessed by measuring SpO2) and in peripheral perfusion; DH was reported as being the principal cause of reduced OUES, and the role of overall muscle strength was not taken into consideration.(7)
The OUES depends on the development of metabolic acidosis, on muscle mass, on oxygen extraction and use, and on physiological pulmonary dead space, which is affected by lung perfusion and structural integrity.(8) It has been suggested that the relationship between muscle strength and OUES depends on muscle mass. However, to our knowledge, this has never been investigated.
Maximal metabolic response to CPET depends on the exercise protocol, encouragement, type of ergometer, peripheral muscle strength, and respiratory muscle strength.(8,9) (Overall) muscle strength is more preserved in the early stages of COPD.(9,10) Therefore, a relevant question is the extent to which a more preserved muscle function, less susceptible to the limiting influence of DH in patients with mild-to-moderate COPD,(11) is a determinant of maximal metabolic response, as evaluated by the lactate level and peak VO2 (VO2peak), in comparison with a submaximal variable reported as being independent of maximal effort, i.e., OUES.
The objective of the present study was to compare the behavior of the OUES with that of the gold standard (VO2peak) in a population of outpatients diagnosed with stage I/II COPD in accordance with the Global Initiative for Chronic Obstructive Lung Disease (GOLD) criteria. We chose to study patients with stage I/II COPD in order to avoid the strong effects of DH on the cardiocirculatory profile of patients with severe COPD. Considering that VO2 efficiency is a variable that depends less on maximal effort during exercise in order to be assessed, we hypothesized that the correlation of this variable with parameters of (peripheral and respiratory) muscle strength at rest and maximal lactate levels was lower.
MethodsThis was a prospective cross-sectional study involving patients recruited from among those being treated at a COPD outpatient clinic or at a smoking outpatient clinic and referred for spirometry. All patients had been diagnosed with stage I/II COPD in accordance with the GOLD criteria(1) and had a post-bronchodilator FEV1/FVC ratio < 70% of predicted and an FEV1 > 50% of predicted, in accordance with reference values for the Brazilian population.(12)
The criteria for patient selection were as follows: being 40 years of age; having a smoking history or being a smoker of 10 pack-years; having experienced no exacerbations in the four weeks preceding the tests; being able to perform exercise tests; being able, from a cardiovascular and metabolic standpoint, to perform the exercise tests; not having coronary/peripheral ischemia, diabetes, hypothyroidism, hyperthyroidism, rheumatic disease, neurological disease, asthma, cognitive deficit, or obstructive sleep apnea; and not using beta-blockers to control mild systemic arterial hypertension. All of the patients gave written informed consent. The study protocol was approved by the Human Research Ethics Committee of the Federal University of Mato Grosso do Sul.
In the first visit, the patients received information on the tests and the following were performed: physical examination; anthropometric data collection; anamnesis (including history of comorbidities and current use of medications); spirometry; measurement of lung volumes and maximal respiratory pressures; handgrip strength (HGS) testing; and arterial blood gas analysis, with appropriate intervals between tests in order to prevent muscle fatigue or discomfort. In the second visit, the patients underwent CPET, capillary blood samples being collected before and after testing. Spirometry and bronchodilator testing were performed in accordance with national guidelines,(13) with a MasterScreen PFT spirometer (Jaeger, Würzburg, Germany). For bronchodilator testing, 400 µg of inhaled albuterol were used. Lung volumes were determined with the same spirometer and the multiple-breath closed-circuit helium dilution technique for calculating functional residual capacity in accordance with national guidelines and reference values for the Brazilian population.(13,14)
Maximal respiratory pressures were measured in accordance with national guidelines,(13) with a digital manometer (model MVD 300; Globalmed, Porto Alegre, Brazil), the signals being read and recorded through individual ducts and precision of 1 cmH2O. A 2-mm hole in the circuit prevented false measurements due to involuntary contractions of the cheeks. The results were provided in cmH2O, and the graphs obtained gave us an idea of the quality of the test. Before the actual test, each patient performed 2-3 measurements in order to become familiar with it. The predicted values were those of Neder et al.(15)
A dynamometer (Jamar; Preston, Jackson, MI, USA) was used in order to measure HGS. The patients were placed in a sitting position, with the arm adducted and parallel to the trunk, the elbow flexed at 90°, and the forearm and wrist in a neutral position. Three measurements were performed, at least 1 min apart, alternating between the dominant and non-dominant arms; the highest value was recorded.(16) Reference values for the Brazilian population were derived from those of Novaes et al.(17) In order to measure blood gases and arterial blood pH, we used an ABL 5 gas analyzer (Radiometer, Copenhagen, Denmark) under anaerobic conditions, through radial artery puncture, in accordance with international recommendations.(18)
For CPET, we used the criteria and protocols recommended in national guidelines.(13) The test was performed in a metabolic system (Oxycon Delta system; Jaeger, Würzburg, Germany) with a mixing chamber for proportional ergospirometry. The system was connected to a program (OxyconLAB; Jaeger) and a cycle ergometer (ER 900; ergoline GmbH., Bitz, Germany) with electromagnetic brakes, the workload and protocol being generated by the program. Calibration was performed before each test, in accordance with the manufacturer protocols, and biological calibration was performed every three months. We defined VO2peak as the mean of the last 15 s at peak exertion. Symptom-limited maximal exercise tolerance was defined as intolerable shortness of breath or leg fatigue preventing cycling at > 50 breaths/min. Maximal voluntary ventilation (MVV) was estimated by multiplying FEV1 by 37.5.(15) The anaerobic threshold (AT) was determined by the V-slope method.(19) The test protocol (incremental ramp protocol) was individualized on the basis of the Wasserman formula to increase the workload and on the basis of the predicted maximal VO2,(20) the predicted value being based on the reference values for the Brazilian population proposed by Neder et al.(21) Lactate was determined from a fingertip blood sample by reflectance photometry (660 nm) with a portable system (Accutrend Lactate; Roche, Mannheim, Germany).
The results are expressed as mean ± SD, and the normality of data distribution was evaluated by the Kolmogorov-Smirnov test. Correlations among variables were established by Pearson's correlation coefficient. In order to calculate the OUES, we log-transformed VE (log10VE) and performed a simple regression analysis:
VO2 = a × logVE + b
where "a" represents the OUES in L and "b" is a constant.
For this calculation, we used the entire period of exercise, because it has been shown that the logarithmic transformation of VE reduces the slope because of the anaerobic wave.(2) Maximal metabolic response was measured by VO2peak and by the ratio between lactate at peak exertion (LACpeak), in mM, and maximum power, in W. Maximum power was used in order to adjust for possible gender bias in aerobic capacity.
ResultsThe data related to anthropometric variables, spirometry, lung volumes, muscle strength, and blood gas analysis in the study population are shown in Table 1. The study sample consisted of 21 patients (15 men), the mean age being 60.7 ± 7.8 years. Lung volumes, peripheral muscle strength, and respiratory muscle strength (all in % of predicted) were within the normal range, as were PaO2 and PaCO2 at rest.
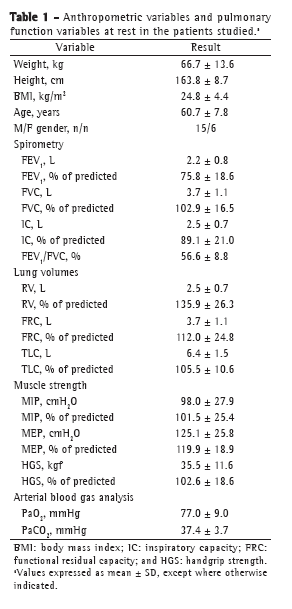
The means of the metabolic, ventilatory, and hemodynamic values selected at peak exertion are shown in Table 2. The values of VO2peak, maximum power, and maximum HR, all in% of predicted, were consistent with those expected for a population of patients with mild-to-moderate COPD. Only 2 patients showed a > 4% drop in SpO2 during exercise, and 7 patients showed evidence of airflow limitation. Of those 7 patients, 1 was female (VE/MVV > 0.75) and 6 were male (VE/MVV > 0.85).
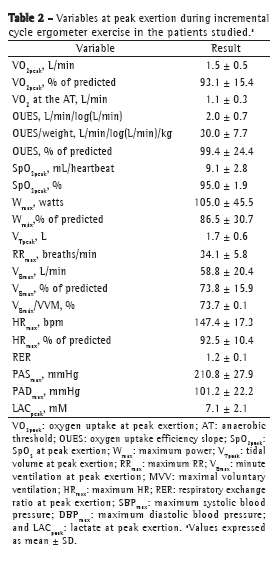
It was impossible to determine the AT in 1 patient only (FEV1 = 51.2% of predicted). For the remaining patients, the mean VO2 at the AT was 1.1 ± 0.3 L/min, its correlation with the OUES being unusually high (r = 0.899; p < 0.0001). The OUES correlated significantly with VO2peak (r = 0.747; p < 0.0001). Although there are no reference values for the OUES in the Brazilian population, the mean OUES found in the present study (99.4 ± 24.4% of predicted) was consistent with the normal range reported in one study.(21)
Table 3 presents the coefficients of correlation of variables at rest/during exercise with peripheral/respiratory muscle strength variables in the study population. As can be seen in Table 3, OUES and weight-indexed OUES, respectively, showed a weaker correlation with peripheral muscle strength (r = 0.453, p < 0.05; and r = 0.345, p > 0.05) and MIP (r = 0.532 and r = 0.454; p < 0.05 for both) than did VO2peak and weight-indexed VO2peak, respectively (r = 0.734, p < 0.0001 and r = 0.641, p < 0.01; and r = 0.733, p < 0.0001 and r = 0.681, p < 0.01; Figure 1).
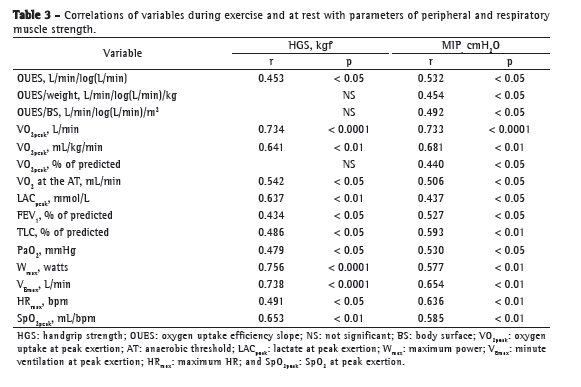
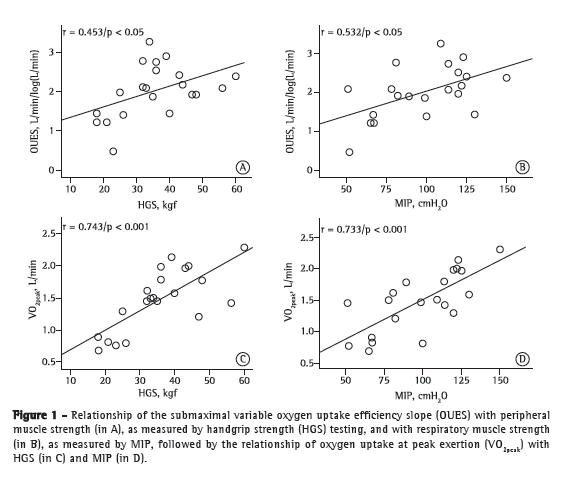
We found no significant correlation between OUES and LACpeak. However, we found a significant negative correlation between OUES and LACpeak corrected for maximum exercise power (r = −0.605 and p = 0.004; Figure 2), as well as between LACpeak/maximum power and VO2peak (r = −0.720 and p < 0.0001; Figure 2). In addition, we found moderate correlations between HGS and SpO2 at peak exertion (r = 0.653 and p < 0.01; Table 3) and between SpO2 at peak exertion and MIP (r = 0.585 and p < 0.01; Table 3).
DiscussionThe principal finding of the present study is that VO2peak correlates better with peripheral and respiratory muscle strength than with the OUES in patients with mild-to-moderate COPD. In addition, the mean OUES was found to be normal for this group of patients with mild-to-moderate COPD, although one third of the patients showed some degree of airflow limitation during cycle ergometer exercise. Some of our findings are consistent with previous findings showing generally preserved aerobic capacity (VO2peak) in patients with mild-to-moderate COPD.(22)
Some of the advantages of using the OUES in healthy and ill individuals alike are as follows:
There are no significant differences between the results obtained with a cycle ergometer and those obtained with a treadmill.(3)
Reproducibility studies have found an extremely high coefficient of variation for the submaximal variable VO2 at the AT, which varies according to the calculation method used.(8)
The OUES can be obtained with submaximal exercise, showing high correlation with VO2peak.(8,23,24)
The OUES has been shown to be more stable and reproducible than VO2peak, especially after training and rehabilitation.(8)
However, further studies involving patients with COPD are needed.
Regarding the correlation between OUES and VO2peak, our findings are consistent with those reported in a recent review of the OUES, in which the correlation coefficient was found to range from 0.72 to 0.96 across the studies.(8) However, our finding of an unexpectedly strong correlation between VO2 at the AT and OUES is at odds with those of that review, in which the correlation coefficient was found to range from 0.66 to 0.78 across the studies.(8) Possible explanations for this difference include the type of disease studied, the size of the clinical population, and the criteria for determining VO2 at the AT. In this aspect, because OUES is a submaximal measurement, it has the advantage of reflecting the effects of metabolic acidosis and those of physiological pulmonary dead space, whereas VO2 at the AT primarily reflects the distribution of blood flow to the exercising muscles.(2)
The OUES estimates the efficiency of ventilation in relation to VO2, a higher slope translating to greater ventilatory efficiency. In fact, the OUES reflects the absolute rate of increase in VO2 for a tenfold increase in ventilation.(25) The increase in ventilation in patients with mild-to-moderate COPD is also limited by DH.(26,27) Because a greater ventilatory demand is one of the determinants of DH, it is reasonable to assume that a proportion of patients in the present study showed DH during exercise, given that 33% of the patients showed evidence of airflow limitation (VE/MVV > 0.75). In theory, this can lead to decreased venous return, worsening the uneven distribution of ventilation, as has been suggested to occur in patients with mild COPD.(26) This could generate a ventilatory inefficiency that is disproportionate to the relatively preserved muscle strength of these patients. However, we do not believe that this effect was significant in our study sample; the mean OUES was found to be within the normal range, and only 1 patient had SpO2 < 80% of the predicted value. Therefore, if any given patient in our sample did present with DH, it probably did not affect the OUES significantly, unlike what was seen in patients with moderate-to-severe COPD, in whom DH caused a significant reduction in the OUES.(7)
Although we found a high correlation between LACpeak and variables such as VO2peak and maximum power, we found no significant correlation between maximal lactate levels and OUES. There are two possible explanations for this finding. First, because OUES is a submaximal variable, it might represent an independent linear system, as was demonstrated for other submaximal variables, such as the variable tau in the analysis of oxygen deficit and the primary gain of aerobic efficiency (VO2/power) during exercise testing at a constant workload, these variables having been found to be independent of the maximal lactate levels achieved.(28) Second, the OUES might be significantly lower in females.(29) The latter hypothesis gains strength when LACpeak is adjusted for maximum power, LACpeak thus correlating significantly with the OUES. This indicates that the oxidative capacity of the muscles adjusted for the workload, at maximal metabolic rate during exercise, significantly reflects oxygen uptake efficiency. The small number of individuals in our sample does not allow us to perform a separate analysis of the possible causes of this difference.
We found a correlation between peripheral muscle strength (HGS) and SpO2 at peak exertion. This finding is consistent with those reported by Celli et al.(30) in patients with moderate-to-severe COPD at peak exertion. Those authors also found a significant correlation between muscle strength and SpO2 at rest, suggesting a more direct relationship between loss of peripheral muscle function and loss of cardiac muscle function. Although COPD was more severe in the patients investigated in the abovementioned study, mean SpO2 values were higher in that study than in ours (10.6 ± 3.7 mL/heartbeat vs. 9.1 ± 2.8 mL/heartbeat), as were mean HGS values (37.8 ± 7.5 kgf vs. 35.5 ± 11.6 kgf). This can be partly attributed to the fact that that study included male patients only.
One of the limitations of the present study is the small number of patients in our clinical sample, which was partly due to the difficulty in finding patients in the early stages of the disease for inclusion in the study. Another limitation is the type of peripheral muscle strength studied. Although there is a controversial correlation between muscle strength as assessed by HGS testing and muscle strength as assessed by isokinetic dynamometry of leg muscles, arm muscle strength is more preserved in patients with COPD and does not reflect well the potential impact of the various etiologic factors involved in muscle function loss. Nevertheless, changes in arm muscle strength limit activities of daily living in such patients and are involved in ventilatory adjustments; in addition, for an equal work rate, ventilation and VO2 are generally higher for arm exercises than for leg exercises.(10) Because our laboratory is not equipped for isokinetic dynamometry, we were unable to evaluate leg muscle strength. We also recognize that the inclusion of female patients in the present study posed a challenge for the analysis of the results. However, it is known that the respiratory pattern of healthy females during incremental exercise is a tachypneic pattern, which therefore results in greater ventilatory inefficiency during submaximal exercise.(20) Further studies are needed in order to determine whether greater ventilatory inefficiency during submaximal exercise in females is accompanied by lower OUES values.
On the basis of the results of the present study, we conclude that the correlations of OUES with peripheral muscle strength and respiratory muscle strength are weaker than are those of VO2peak with peripheral muscle strength and respiratory muscle strength in patients with mild-to-moderate COPD. The high correlation between OUES and VO2peak suggests that the former is a physiological variable that is more dependent on other determinants of VO2, such as gas exchange and lung perfusion, and less dependent on the baseline muscle strength of individuals.
References1. Rabe KF, Hurd S, Anzueto A, Barnes PJ, Buist SA, Calverley P, et al. Global strategy for the diagnosis, management, and prevention of chronic obstructive pulmonary disease: GOLD executive summary. Am J Respir Crit Care Med. 2007;176(6):532-55. PMid:17507545. http://dx.doi.org/10.1164/rccm.200703-456SO
2. Baba R, Nagashima M, Goto M, Nagano Y, Yokota M, Tauchi N, et al. Oxygen uptake efficiency slope: a new index of cardiorespiratory functional reserve derived from the relation between oxygen uptake and minute ventilation during incremental exercise. J Am Coll Cardiol. 1996;28(6):1567-72. http://dx.doi.org/10.1016/S0735-1097(96)00412-3
3. Sun XG, Hansen JE, Stringer WW. Oxygen uptake efficiency plateau: physiology and reference values. Eur J Appl Physiol. 2012;112(3):919-28. PMid:21695524. http://dx.doi.org/10.1007/s00421-011-2030-0
4. Lindberg A, Larsson LG, Rönmark E, Lundbäck B. Co-morbidity in mild-to-moderate COPD: comparison to normal and restrictive lung function. COPD. 2011;8(6):421-8. PMid:22149402. http://dx.doi.org/10.3109/15412555.2011.629858
5. Van Laethem C, Bartunek J, Goethals M, Nellens P, Andries E, Vanderheyden M. Oxygen uptake efficiency slope, a new submaximal parameter in evaluating exercise capacity in chronic heart failure patients. Am Heart J. 2005;149(1):175-80. PMid:15660050. http://dx.doi.org/10.1016/j.ahj.2004.07.004
6. Arena R, Myers J, Abella J, Peberdy MA, Bensimhon D, Chase P, et al. The influence of body mass index on the oxygen uptake efficiency slope in patients with heart failure. Int J Cardiol. 2008;125(2):270-2. PMid:18234369. http://dx.doi.org/10.1016/j.ijcard.2007.11.013
7. Tzani P, Aiello M, Elia D, Boracchia L, Marangio E, Olivieri D, et al. Dynamic hyperinflation is associated with a poor cardiovascular response to exercise in COPD patients. Respir Res. 2011;12:150. PMid:22074289. PMCid:3225311. http://dx.doi.org/10.1186/1465-9921-12-150
8. Akkerman M, van Brussel M, Hulzebos E, Vanhees L, Helders PJ, Takken T. The oxygen uptake efficiency slope: what do we know? J Cardiopulm Rehabil Prev. 2010;30(6):357 73. PMid:20724931.
9. Miranda EF, Malaguti C, Corso SD. Peripheral muscle dysfunction in COPD: lower limbs versus upper limbs. J Bras Pneumol. 2011;37(3):380-8. PMid:21755195. http://dx.doi.org/10.1590/S1806-37132011000300016
10. Skeletal muscle dysfunction in chronic obstructive pulmonary disease. A statement of the American Thoracic Society and European Respiratory Society. Am J Respir Crit Care Med. 1999;159(4 Pt 2):S1-40. PMid:10194189.
11. Freitas CG, Pereira CA, Viegas CA. Inspiratory capacity, exercise limitation, markers of severity, and prognostic factors in chronic obstructive pulmonary disease. J Bras Pneumol. 2007;33(4):389-96. PMid:17982530. http://dx.doi.org/10.1590/S1806-37132007000400007
12. Pereira CA, Sato T, Rodrigues SC. New reference values for forced spirometry in white adults in Brazil. J Bras Pneumol. 2007;33(4):397-406. PMid:17982531. http://dx.doi.org/10.1590/S1806-37132007000400008
13. Sociedade Brasileira de Pneumologia e Tisiologia. Diretrizes para Testes de Função Pulmonar. J Pneumol. 2002;28(Suppl 3):S1-S238.
14. Neder JA, Andreoni S, Castelo-Filho A, Nery LE. Reference values for lung function tests. I. Static volumes. Braz J Med Biol Res. 1999;32(6):703-17. PMid:10412549.
15. Neder JA, Andreoni S, Lerario MC, Nery LE. Reference values for lung function tests. II. Maximal respiratory pressures and voluntary ventilation. Braz J Med Biol Res. 1999;32(6):719-27. http://dx.doi.org/10.1590/S0100-879X1999000600007
16. Caporrino FA, Faloppa F, Santos JB, Réssio C, Soares FH, Nakachima LR, et al. Estudo populacional da força de preensão palmar com dinamômetro Jamar. Rev Bras Ortop Traumatol. 1998;33(2):150-4.
17. Novaes RD, Miranda AS, Silva JO, Tavares BV, Dourado VZ. Equações de referência para a predição da força de preensão manual em brasileiros de meia idade e idosos. Fisioter Pesq. 2009;16(3):217-22. http://dx.doi.org/10.1590/S1809-29502009000300005
18. Clinical and Laboratory Standards Institute. Blood gas and pH analysis and related measurements; approved guideline. Wayne: Clinical and Laboratory Standards Institute; 2001.
19. Beaver WL, Wasserman K, Whipp BJ. A new method for detecting anaerobic threshold by gas exchange. J Appl Physiol. 1986;60(6):2020-7. PMid:3087938.
20. Neder JA, Nery LE, editors. Fisiologia Clínica do Exercício. Teoria e Prática. São Paulo: Artes Médicas; 2003.
21. Neder JA, Nery LE, Castelo A, Andreoni S, Lerario MC, Sachs A, et al. Prediction of metabolic and cardiopulmonary responses to maximum cycle ergometry: a randomised study. Eur Respir J. 1999;14(6):1304-13. PMid:10624759. http://dx.doi.org/10.1183/09031936.99.14613049
22. Pinto-Plata VM, Celli-Cruz RA, Vassaux C, Torre-Bouscoulet L, Mendes A, Rassulo J, et al. Differences in cardiopulmonary exercise test results by American Thoracic Society/European Respiratory Society-Global Initiative for Chronic Obstructive Lung Disease stage categories and gender. Chest. 2007;132(4):1204-11. PMid:17934113. http://dx.doi.org/10.1378/chest.07-0593
23. Hollenberg M, Tager IB. Oxygen uptake efficiency slope: an index of exercise performance and cardiopulmonary reserve requiring only submaximal exercise. J Am Coll Cardiol. 2000;36(1):194-201.
24. Mueller PT, Bollmann T, Gläser S, Czekay S, Kähler C, Ewert R. VO2 Kinetik und VO2 Effizienz slope bei Patienten mit Idiopathischer Pulmonaler Arterieller Hypertonie (IPAH). Pneumologie. 2011;65:P388. http://dx.doi.org/10.1055/s-0031-1272107
25. Baba R. The oxygen uptake efficiency slope and its value in the assessment of cardiorespiratory functional reserve. Congest Heart Fail. 2000;6(5):256-8. PMid:12189286. http://dx.doi.org/10.1111/j.1527-5299.2000.80164.x
26. Ofir D, Laveneziana P, Webb KA, Lam YM, O'Donnell DE. Mechanisms of dyspnea during cycle exercise in symptomatic patients with GOLD stage I chronic obstructive pulmonary disease. Am J Respir Crit Care Med. 2008;177(6):622-9. PMid:18006885. http://dx.doi.org/10.1164/rccm.200707-1064OC
27. Babb TG, Viggiano R, Hurley B, Staats B, Rodarte JR. Effect of mild-to-moderate airflow limitation on exercise capacity. J Appl Physiol. 1991;70(1):223-30. PMid:2010380.
28. Barstow TJ, Casaburi R, Wasserman K. O2 uptake kinetics and the O2 deficit as related to exercise intensity and blood lactate. J Appl Physiol. 1993;75(2):755-62. PMid:8226479.
29. Pogliaghi S, Dussin E, Tarperi C, Cevese A, Schena F. Calculation of oxygen uptake efficiency slope based on heart rate reserve end-points in healthy elderly subjects. Eur J Appl Physiol. 2007;101(6):691-6. PMid:17717680. http://dx.doi.org/10.1007/s00421-007-0545-1
30. Cortopassi F, Divo M, Pinto-Plata V, Celli B. Resting handgrip force and impaired cardiac function at rest and during exercise in COPD patients. Respir Med. 2011;105(5):748-54. PMid:21251806. http://dx.doi.org/10.1016/j.rmed.2010.12.011
* Study carried out at the Federal University of Mato Grosso do Sul, Campo Grande, Brazil.
Correspondence to: Paulo de Tarso Guerrero Müller. Avenida Filinto Müller, s/n, Campus da Universidade Federal de Mato Grosso do Sul, Faculdade de Medicina, Vila Ipiranga, CEP 79070-900, Campo Grande, MS, Brasil.
Tel. 55 67 3345-3149 or 55 67 9291-0441. E-mail: paulo.muller@ufms.br
Financial support: None.
Submitted: 8 March 2012. Accepted, after review: 15 August 2012.
About the authorsPaulo de Tarso Guerrero Müller
Professor. Federal University of Mato Grosso do Sul School of Medicine,
Campo Grande, Brazil.
Carlos Alberto de Assis Viegas
Professor. University of Brasília School of Medicine, Brasília, Brazil.
Luiz Armando Pereira Patusco
Professor. Federal University of Mato Grosso do Sul School of Medicine, Campo Grande, Brazil.