ABSTRACT
Objective: To identify associations between genetic polymorphisms (in the MBL2, TGF-β1 and CD14 genes) and the severity of the lung disease in patients with cystic fibrosis (CF), as well as between the presence of ΔF508 alleles and lung disease severity in such patients. Methods: This was a cross-sectional cohort study, based on clinical and laboratory data, involving 105 patients with CF treated at a university hospital in the 2005-2006 period. We included 202 healthy blood donors as controls for the determination of TGF-β1 and CD14 gene polymorphisms. Polymorphisms in the MBL2 and TGF-β1 genes at codon 10, position +869, were genotyped using the allele-specific PCR technique. The C-159T polymorphism in the CD14 gene was genotyped using PCR and enzymatic digestion. Results: Of the 105 CF patients evaluated, 67 presented with severe lung disease according to the Shwachman score. The MBL2 gene polymorphisms were not associated with disease severity in the CF patients. Analysis of the T869C polymorphism in the TGF-β1 gene showed an association only between TC heterozygotes and mild pulmonary disease. Although patients presenting the TT genotype of the C159T polymorphism in the CD14 gene predominated, there was no significant difference regarding lung disease severity. Conclusions: There was an association between the TC genotype of the T869C polymorphism (TGF-β1) and mild pulmonary disease in CF patients. In the CD14 gene, the TT genotype seems to be a risk factor for pulmonary disease but is not a modulator of severity. We found no association between being a ΔF508 homozygote and presenting severe lung disease.
Keywords:
Cystic fibrosis; Polymorphism, genetic; Severity of illness index; Mannose-binding lectin; Transforming growth factor beta.
RESUMO
Objetivo: Verificar a correlação entre os polimorfismos dos genes MBL2, TGF-β1 e CD14 com a gravidade da doença pulmonar em pacientes com fibrose cística (FC), bem como correlacionar a presença dos alelos ΔF508 com a gravidade da doença naqueles pacientes. Métodos: Estudo clínico-laboratorial, de corte transversal, com 105 pacientes fibrocísticos de um hospital universitário em 2005-2006. Foram analisados 202 doadores de sangue saudáveis como controles para a pesquisa dos polimorfismos no gene TGF-β1 e CD14. A análise de polimorfismos nos genes MBL2 e TGF-β1 no códon 10, posição +869, foi realizada pela técnica da PCR alelo-específica. A genotipagem do polimorfismo C-159T no gene CD14 foi realizada através de PCR e digestão enzimática. Resultados: Dos 105 pacientes com FC avaliados, 67 apresentavam doença pulmonar grave segundo o escore de Shwachman. Os polimorfismos do gene MBL2 não foram associados com a gravidade da doença nos fibrocísticos. A análise do polimorfismo T869C no gene TGF-β1 mostrou somente uma associação entre o heterozigoto TC com doença pulmonar leve. Para o polimorfismo C-159T no gene CD14, obtivemos um predomínio de pacientes com o genótipo TT, mas não houve diferença significativa com relação à gravidade do quadro pulmonar. Conclusões: Houve associação entre o genótipo TC do polimorfismo T869C (TGF-β1) e o quadro pulmonar leve nos fibrocísticos. No gene CD14, o genótipo TT parece ser um fator de risco para o quadro pulmonar, mas não um fator modulador da gravidade. Não existiu associação entre pacientes homozigotos para a mutação ΔF508 e a gravidade do quadro pulmonar.
Palavras-chave:
Fibrose cística; Polimorfismo genético; Índice de gravidade de doença; Lectina de ligação a manose; Fator transformador de crescimento beta.
IntroduçãoA fibrose cística (FC) é uma doença autossômica recessiva causada por mais de 1.600 mutações no gene que codifica a proteína cystic fibrosis transmembrane conductance regulator (CFTR, reguladora de condutância transmembrana da fibrose cística), localizado no braço longo do cromossomo 7. Essas mutações são distribuídas em seis classes. Existem fenótipos clássicos e atípicos desta doença, dependentes principalmente da classe e do tipo de mutação. A taxa de incidência é de 1/2.500 recém-nascidos, e a de portadores é de 1/25.(1-3)
Estudos populacionais, com grande número de fibrocísticos, comprovam a relação genótipo-fenótipo, principalmente para as manifestações pancreáticas, mas muito pouco para as pulmonares. Portanto, as características genéticas da relação genótipo-fenótipo nas manifestações pulmonares permanecem pouco esclarecidas. Sabe-se que mesmo indivíduos homozigotos para a mutação de maior prevalência (ΔF508) apresentam grande variabilidade no comprometimento e na evolução da doença pulmonar.(4)
Embora influências ambientais possam interferir nas manifestações clínicas pulmonares, tem sido descrita a possibilidade de suma variação genética adicional, como a presença de genes modificadores, contribuindo para a expressão clínica final em cada paciente. Alguns autores têm relatado que polimorfismos em genes que não o CFTR podem modificar a gravidade da doença pulmonar na FC.(5)
Um estudo com gêmeos monozigóticos mostrou uma concordância maior com relação à gravidade da doença pulmonar quando comparada à gravidade em gêmeos dizigóticos, sugerindo uma forte contribuição genética na variabilidade da gravidade da doença pulmonar nos fibrocísticos.(6)
Genes modificadores fora do gene CFTR podem influenciar a gravidade do fenótipo dos fibrocísticos através de vários mecanismos: podem modular o fenótipo alternando a condução de cloro; podem regular o splicing e a expressão do gene CFTR; e podem modular a susceptibilidade à infecção por bactérias e a resposta inflamatória nos pulmões. Além disso, o quadro pulmonar dos fibrocísticos pode ser modificado por genes associados com clearance mucociliar e com os danos e reparos do tecido epitelial.(7)
O conceito de múltiplos modificadores genéticos na doença mendeliana, como na FC, é diferente do conceito de múltiplos variantes genéticos na doença não-mendeliana, como na asma. Nas doenças genéticas complexas, como na asma, variantes genéticas múltiplas interagem entre si e com o ambiente, causando a doença. Ao contrário, a FC, por ser uma doença mendeliana, é causada por mutações no gene CFTR, e há variações genéticas não ligadas ao gene CFTR que podem ser desfavoráveis ou benéficas e que modificam a gravidade do fenótipo em conjunto com fatores ambientais. De fato, polimorfismos genéticos, com ou sem efeitos em sujeitos saudáveis, podem ser modificadores na FC.(4)
A identificação da consequência da ação de genes modificadores permitirá um melhor entendimento dos aspectos fisiopatológicos, da correlação genótipo-fenótipo e maximizará o tratamento da FC.(8)
Dentre os genes modificadores encontram-se:
MBL2: Localizado no cromossomo 10, no braço q11.2-q21. Codifica a proteína mannose-binding lectin (MBL, lectina ligadora da manose). É uma proteína plasmática com papel importante no sistema de defesa inato, que constitui o primeiro componente de ativação da via das lectinas do sistema complemento e atua na neutralização de microorganismos patogênicos por um mecanismo independente de anticorpo.(9) A sua deficiência esta relacionada com a função pulmonar diminuída dos fibrocísticos.
TGF-β1: O gene TGF-β1 tem sido mapeado no cromossomo 19q13.1-q13.3. Esse gene é expresso em células endoteliais, hematopoiéticas e células de tecidos relacionados. O gene TGF-β1 codifica a proteína transforming growth factor β1 (TGF- β1, fator transformador de crescimento β1), a qual é membro de uma família de fatores de crescimento e diferenciação, com múltiplas funções em uma variedade de diferentes sistemas de órgãos. A proteína TGF-β é notável por sua capacidade de modular uma variedade de funções celulares, incluindo a proliferação celular, diferenciação e apoptose in vivo e in vitro.(10) Tanto a baixa quanto a alta expressão desta proteína podem causar alterações no trato respiratório.
CD14: O gene CD14 está localizado no cromossomo 5q31.1, com 3.900 bp, apresenta dois éxons e codifica uma proteína de 375 aminoácidos, sendo expresso no limite da membrana de macrófagos, monócitos e neutrófilos. Funciona como receptor para lipopolissacarídeos, componente da membrana externa de bactérias gram negativas. É um elemento constitutivo da parede celular de Pseudomonas aeruginosa, que possui grande poder imunogênico.(1,11)
A baixa expressão de CD14 tem sido relacionada à colonização precoce de bactérias nos pulmões, incluindo a P. aeruginosa.
O objetivo desse estudo foi verificar a associação entre os polimorfismos do éxon 1 (códons 52, 54 e 57) e a região promotora (haplótipos HY, LY e LX) do gene MBL2, o polimorfismo T869C no gene TGF-β1 e o polimorfismo C-159T no gene CD14 com a gravidade do quadro pulmonar de pacientes com FC, assim como analisar a presença dos alelos ΔF508 em relação à gravidade do quadro pulmonar nos pacientes fibrocísticos.
MétodosRealizou-se um estudo clínico-laboratorial, de corte transversal, com os pacientes do Ambulatório de Fibrose Cística do Departamento de Pediatria junto ao Hospital de Clínicas da Universidade Estadual de Campinas (Unicamp), entre 2005 e 2006. Foram incluídos no estudo todos os pacientes que estavam em seguimento nesse período e que tinham o diagnóstico de FC, confirmado por história clínica compatível, por pelo menos dois testes do suor com valores de cloro igual ou superiores a 60 mEq/L realizados através do estímulo do suor por iontoforese com pilocarpina(12) e/ou por confirmação da mutação genética.
Foram analisados 105 pacientes fibrocísticos, sendo que 67 apresentavam a classificação clínica de quadro pulmonar. Foram incluídos 202 controles (indivíduos saudáveis, doadores de sangue) para os polimorfismos no gene TGF-β1 e CD14.
O estudo foi aprovado pelo Comitê de Ética em Pesquisa da Faculdade de Ciências Médicas da Unicamp, e todos os responsáveis pelos pacientes assinaram o termo de consentimento esclarecido antes do início do estudo.
Os critérios clínicos analisados incluíam as manifestações pulmonares, as manifestações digestivas e o escore de Shwachman (ES).(13) A avaliação laboratorial incluiu testes de função pulmonar, dosagem de sódio e cloro no suor, radiografia de tórax e TCAR de tórax. O ES avalia a atividade física, o exame físico, a nutrição e o quadro radiológico. Para cada item, a pontuação máxima é de 25 pontos; quanto menor o valor do escore, pior o quadro clínico do paciente. O escore é graduado em excelente (86-100), bom (71-85), médio (56-70), moderado (41-55) e grave (40 ou menos), conforme o número total de pontos. Todos os pacientes foram previamente genotipados quanto ao gene CFTR pela equipe do Laboratório de Genética Molecular do Hospital Universitário. O DNA foi extraído e, através da técnica de PCR, regiões específicas foram amplificadas para que pudessem ser analisadas para as seguintes mutações: ΔF508, G542X, N1303K, G551D e R553X.
Para a análise da MBL, foi realizada a extração de DNA de leucócitos do
sangue periférico.(14) Após a extração de DNA, o método utilizado foi a sequência de primers específicos, através do qual foram realizadas várias reações de amplificação, cada uma delas contendo um iniciador capaz de detectar um alelo ou grupo de alelos. Através da PCR de sequência de primers específicos, determinamos a genotipagem de 105 indivíduos para as mutações conhecidas na região promotora H e L na posição −550 (G-C)-localizado 550 bp antes do sítio de início da transcrição, onde ocorre a troca da guanina pela citosina-e X e Y, na posição −221 (G-C)-localizado 221 bp antes do sítio de início da transcrição, onde ocorre a troca da guanina pela citosina. Os polimorfismos −550 e −221 na região promotora formam os haplótipos HY, LY e LX.
No éxon 1 estão os códons 52, 54 e 57, que originam três variáveis alelos (denominados D, B e C, respectivamente). O alelo normal tem sido denominado A, e os variáveis alelos D, B e C são classificados como O. As três mutações de ponto ocorrem nos nucleotídeos 223 (C-T)-troca da citosina pela timina-230 (G-A)-troca da guanina pela adenina-e 239 (G-A)-troca da guanina pela adenina-nos alelos D, B e C, respectivamente.
Para a análise do gene TGF-β1, foi realizada a extração de DNA de leucócitos do sangue periférico(14). Após a extração de DNA, identificamos o polimorfismo no gene do TGF-β1, localizado no códon 10, posição +869 (T-C)-troca da timina pela citosina-através da técnica denominada sistema de mutação refratário à amplificação.
Para a análise do gene CD14, foi realizada a extração de DNA de leucócitos do sangue periférico.(14) Após a extração de DNA, a genotipagem do polimorfismo CD14 (C-159T, troca da citosina pela timina) foi realizada.
Os métodos, as sequências de primers e as enzimas de restrições utilizadas, bem como o tamanho dos fragmentos gerados pelos polimorfismos dos genes MBL2, TGF-β1 e CD14, estão descritos na Tabela 1.(15-17)
A análise dos resultados e das associações entre as variáveis dos pacientes fibrocísticos e as do grupo controle foram feitas através do teste do qui-quadrado e OR. A diferença entre os grupos foi estatisticamente significativa quando o valor do teste aplicado foi p < 0,05. O programa estatístico utilizado foi o Epi Info 2000, versão 1.1.2.
ResultadosForam analisados 105 pacientes com FC (53 masculinos e 52 femininos) em seguimento no Ambulatório Multidisciplinar de Fibrose Cística do Departamento de Pediatria da Unicamp. Em 67 pacientes, foi aplicado o ES. Os pacientes apresentaram média de idade de 7,8 ± 0,71 anos, sendo que 101 (96%) eram caucasoides e 4 (4%) pardos.
Foram analisados 202 controles doadores de sangue-117 homens (58%) e 85 mulheres (42%)-para os polimorfismos no gene TGF-β1 e CD14, com média de idade de 34 ± 11,3 anos, sendo 160 brancos (79,2%), 41 negroides (20,3%) e 1 de etnia oriental (0,5%).
Estudamos a presença dos alelos ΔF508 em relação à gravidade do quadro pulmonar. A comparação da presença ou da ausência de dois alelos ΔF508 em relação à gravidade do quadro pulmonar mostrou resultado estatisticamente significativo para os pacientes graves. Nestes pacientes, a ausência dos alelos ΔF508 representou um fator de risco (p = 0,01; OR = 16,29; variação, 1,43-787,09; Tabela 2).
Na análise dos polimorfismos nos códons 52, 54 e 57 (alelos AO) do gene MBL2 em relação à presença dos alelos para mutação ΔF508, a amostra de pacientes fibrocísticos não está em equilíbrio de Hardy-Weinberg (EWH; χ(2)2 = 9,95; p = 0,007). Não houve resultados significativos na análise desses polimorfismos.
Na análise dos polimorfismos H/L e X/Y na região promotora do gene MBL2, em relação à presença dos alelos da mutação ΔF508, a amostra de pacientes fibrocísticos está em EHW (χ(5)2 = 8,82; p = 0,11). Não houve resultados significativos na análise desses polimorfismos.
Na análise do polimorfismo T869C no gene TGF-β1, a amostra de pacientes fibrocísticos não está em EHW (χ(2)2 = 21,24; p = 0,000024). A amostra controle está em EHW (χ(2)2 =10,58; p = 0,005). Quando foi realizada a comparação genotípica dos pacientes fibrocísticos e os do grupo controle, foi observada uma diferença significativa com relação aos genótipos TC e CC, sendo o genótipo TC um fator de risco na amostra (p = 0,01; OR = 2,00; variação, 1,11‑3,61; Tabela 3).
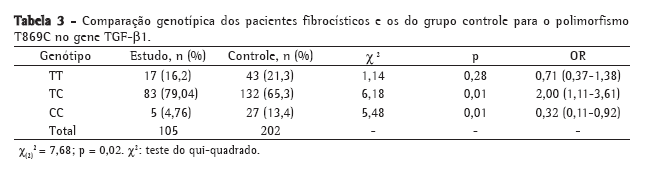
Com relação à distribuição genotípica do polimorfismo T869C no gene TGF-β1 nos indivíduos controle comparados com a gravidade do quadro pulmonar nos fibrocísticos, houve apenas um resultado significativo com relação ao quadro pulmonar leve. O genótipo TC (p = 0,01; OR = 4,07; variação, 1,16-21,78) apresentou um fator de risco para o quadro pulmonar leve nos fibrocísticos (Tabela 4).
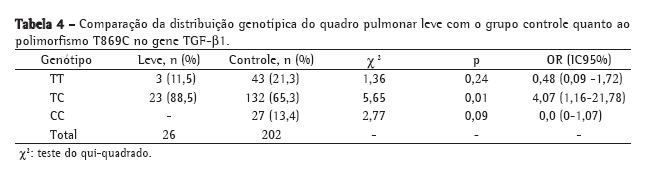
Na análise do polimorfismo C159T no gene CD14, a amostra de pacientes fibrocísticos está em EWH (χ(2)2 = 4,38; p = 0,11). A amostra controle não está em EWH (χ(2)2 = 18,72; p = 0,00008).
Ao realizar a comparação genotípica entre o grupo de pacientes fibrocísticos e o grupo controle quanto ao polimorfismo C159T no gene CD14, foi encontrada diferença significativa com relação ao genótipo TT, sendo que esse genótipo apresentou um fator de risco na amostra, mas não um fator modulador da gravidade do quadro pulmonar (p = 0,001; OR = 4,36; variação, 1,68-12,16; Tabela 5).
DiscussãoEntre as DPOCs, a asma e a FC manifestam-se fenotipicamente como consequência de um componente genético e de outro ambiental que determinam a gravidade e o curso clinico ao longo da vida dos pacientes com estas enfermidades.
Na FC e na asma, foram identificadas mutações em um e em mais de 100 genes, respectivamente, caracterizando a identificação de muitos fenótipos nestas duas DPOCs. Percebe-se, portanto, que a complexidade fenotípica da asma é bem maior do que da FC. Em contrapartida, enquanto a associação de polimorfismos com as manifestações fenotípicas tem sido muito estudada na asma, os estudos são poucos na FC.
Após extensa revisão da literatura, verificamos que este é o primeiro estudo no Brasil a verificar a associação entre os polimorfismos dos genes MBL2, TGF-β1 e CD14 com a gravidade do quadro pulmonar em crianças e adolescentes com FC.
No presente estudo, para os polimorfismos em que a amostra controle não se encontrava em EHW, a explicação provável decorre do fato de que no EHW preconiza-se uma população ideal, sem pressão seletiva. No caso dos polimorfismos estudados, por interferirem em mecanismos relacionados com a inflamação, é possível que determinados genótipos sofram uma pressão seletiva e, com isso, a distribuição genotípica não seguiu o EHW.
Na FC, a doença pulmonar pode ser influenciada por fatores genéticos, fatores ambientais e genes modificadores localizados fora do gene CFTR.(18)
Diferentemente de outros estudos, na análise da gravidade do quadro pulmonar e da presença de alelos ΔF508, verificamos uma diminuição de homozigotos (ΔF508/ΔF508) entre os pacientes com quadro pulmonar grave, demonstrando não existir associação entre ser homozigoto ΔF508 e maior gravidade do quadro pulmonar.
Em nossa casuística de pacientes com FC, os polimorfismos do gene MBL2 não estiveram associados à gravidade do quadro pulmonar. Uma hipótese para os nossos resultados é o fato de que a maioria dos pacientes tinha idade menor que 15 anos. Alguns autores demonstraram que a deficiência de MBL está relacionada à gravidade do quadro pulmonar somente em pacientes fibrocísticos adolescentes com idade maior de 15 anos ou adultos.(19) Nesses pacientes, o hormônio de crescimento pode afetar significantemente o nível de MBL em circulação. Aquele estudo revelou uma importância significativa da idade e do desenvolvimento físico dos pacientes fibrocísticos no entendimento da relação entre MBL e a função pulmonar na FC.(19)
Vários autores relataram que apenas indivíduos fibrocísticos cujo genótipo é OO (homozigotos para os polimorfismos no éxon 1 do gene MBL2), o qual está relacionado à baixa produção da proteína MBL, leva à diminuição da função pulmonar.(20,21) O mesmo não foi observado em outro estudo, no qual os dois genótipos AO (heterozigoto) e OO (homozigoto) do gene MBL2 estavam associados à piora da função pulmonar.(22,23)
Foi demonstrado que crianças com FC colonizadas com P. aeruginosa e que apresentavam a deficiência de MBL tinham uma disfunção pulmonar mais grave em comparação àquelas com níveis intermediários ou altos da proteína MBL presente em circulação.(24) Os autores demonstraram que esses efeitos modulatórios se devem a alta produção da proteína TGF-β, sugerindo uma interação complexa gene-gene entre MBL2 e TGF-β1.(24)
Estudos sobre a deficiência de MBL e a colonização por P. aeruginosa são promissores e precisam ser realizados em nosso meio. Acredita-se que múltiplos fatores genéticos possam influenciar a resposta que a deficiência de MBL desempenha como função devido aos alelos variáveis desta proteína. Sabe-se que a MBL exerce uma ação complexa sobre a resposta inflamatória em nível pulmonar.
Com relação ao polimorfismo T869C no gene TGF-β1, em nosso estudo, encontramos apenas uma associação de heterozigoto TC com o quadro pulmonar leve.
Dois estudos demonstraram que pacientes com FC portadores do genótipo TT (baixa produção da proteína) no códon 10 do gene TGF-β1 apresentavam um risco elevado para a doença pulmonar.(25,26)
Contrariamente, outros autores observaram que o genótipo CC (elevada produção da proteína) é o que apresentava risco elevado para a deterioração da função pulmonar.(5)
Alguns pesquisadores encontraram uma correlação positiva entre diferentes colonizações das vias respiratórias por diferentes bactérias e a produção da proteína TGF-β1.
A produção diminuída ou aumentada da proteína TGF-β1 em pacientes com FC, colonizados, respectivamente, com Burkholderia cepacia e P. aeruginosa, evidenciam a importância da produção dessa citocina regulatória em diferentes colonizações bacterianas e na gravidade da FC.(27)
A variação encontrada nos estudos relacionados aos genótipos de TGF-β1 com a gravidade do quadro pulmonar pode ser explicada pelas diferenças genotípicas, pelo número de pacientes e pelos aspectos ambientais não controlados entre os grupos dos poucos estudos
publicados.
A detecção do polimorfismo C159T no gene CD14, em nosso estudo, revelou uma predominância de fibrocísticos com o genótipo TT (aumento na produção da proteína CD14), mas não houve uma discriminação com relação à gravidade do quadro pulmonar.
Em um estudo, foi demonstrada a associação entre o polimorfismo CD14-159CC e a colonização precoce das vias respiratórias por P. aeruginosa em crianças fibrocísticas. Essas crianças apresentaram níveis diminuídos da proteína CD14 solúvel no plasma, bem como uma resposta pró-inflamatória inadequada.(11)
Embora crianças com níveis elevados da proteína CD14 solúvel no plasma possam ser relativamente protegidas contra a colonização precoce por P. aeruginosa, ao se tornarem colonizadas, elas podem ter uma resposta inflamatória mais intensa.
Como diferenças étnicas e raciais são comuns em sistemas polimórficos, induzindo a expressão do fenótipo clínico em diferentes populações, é possível que resultados diferentes possam ser encontrados em outras populações ou grupos raciais.
Os resultados obtidos nesse estudo permitem concluir que muitas questões ainda permanecem sobre o entendimento da função dos genes modificadores na FC de distintas populações. Portanto, estudos multicêntricos, avaliando um número maior de pacientes, em cada classe de mutação, são necessários para a compreensão dos efeitos dos genes modificadores na FC.
AgradecimentosOs autores agradecem aos membros da equipe multidisciplinar do
Ambulatório de Fibrose Cística do Hospital de Clinicas da Unicamp.
Referências 1. Davies JC, Griesenbach U, Alton E. Modifier genes in cystic fibrosis. Pediatr Pulmonol. 2005;39(5):383-91.
2. Rommens JM, Iannuzzi MC, Kerem B, Drumm ML, Melmer G, Dean M, et al. Identification of the cystic fibrosis gene: chromosome walking and jumping. Science. 1989;245(4922):1059-65.
3. Cystic fibrosis mutation database [homepage on the Internet]. [updated 2007 Mar 02; cited 2008 Jul 1]. Available from: http://www.genet.sickkids.on.ca/cftr/
4. Knowles MR. Gene modifiers of lung disease. Curr Opin Pulm Med. 2006;12(6):416-21.
5. Drumm ML, Konstan MW, Schluchter MD, Handler A, Pace R, Zou F, et al. Genetic modifiers of lung disease in cystic fibrosis. N Engl J Med. 2005;353(14):1443-53.
6. Vanscoy LL, Blackman SM, Collaco JM, Bowers A, Lai T, Naughton K, et al. Heritability of lung disease severity in cystic fibrosis. Am J Respir Crit Care Med. 2007;175(10):1036-43.
7. Slieker MG, Sanders EA, Rijkers GT, Ruven HJ, van der Ent CK. Disease modifying genes in cystic fibrosis. J Cyst Fibros. 2005;4 Suppl 2:7-13.
8. Boyle MP. Strategies for identifying modifier genes in cystic fibrosis. Proc Am Thorac Soc. 2007;4(1):52-7.
9. Guardia A, Lozano F. Mannose-binding lectin deficiencies in infectious and inflammatory disorders. Rev Med Microbiol. 2003;14:41-52.
10. Grande JP. Role of transforming growth factor-beta in tissue injury and repair. Proc Soc Exp Biol Med. 1997;214(1):27-40.
11. Martin AC, Laing IA, Zhang G, Brennan S, Winfield K, Sly PD, et al. CD14 C-159T and early infection with Pseudomonas aeruginosa in children with cystic fibrosis. Respir Res. 2005;6:63.
12. Gibson LE, Cooke RE. A test for concentration of electrolytes in sweat in cystic fibrosis of the pancreas utilizing pilocarpine by iontophoresis. Pediatrics. 1959;23(3):545-9.
13. Santos CIS, Ribeiro JD, Ribeiro AF, Hessel G. Critical analysis of scoring systems used in the assessment of Cystic Fibrosis severity: State of the art. J Bras Pneumol. 2004;30(3):286-98.
14. Woodhead JL, Fallon R, Figuered H, Longdale J, Malcom AD. Alternative methodology of gene diagnosis. In: Davies KE, editor. Human genetic diseases-a practical approach. Oxford: IRL Press Limited; 1986. p. 51-64.
15. Steffensen R, Thiel S, Varming K, Jersild C, Jensenius JC. Detection of structural gene mutations and promoter polymorphisms in the mannan-binding lectin (MBL) gene by polymerase chain reaction with sequence-specific primers. J Immunol Methods. 2000;241(1-2):33-42.
16. Perrey C, Turner SJ, Pravica V, Howell WM, Hutchinson IV. ARMS-PCR methodologies to determine IL-10, TNF-alpha, TNF-beta and TGF-beta 1 gene polymorphisms. Transpl Immunol. 1999;7(2):127-8.
17. Koppelman GH, Reijmerink NE, Colin Stine O, Howard TD, Whittaker PA, Meyers DA, et al. Association of a promoter polymorphism of the CD14 gene and atopy. Am J Respir Crit Care Med. 2001;163(4):965-9.
18. Mahadeva R, Lomas DA. Secondary genetic factors in cystic fibrosis lung disease. Thorax. 2000;55(6):446.
19. Muhlebach MS, MacDonald SL, Button B, Hubbard JJ, Turner ML, Boucher RC, et al. Association between mannan-binding lectin and impaired lung function in cystic fibrosis may be age-dependent. Clin Exp Immunol. 2006;145(2):302-7.
20. Gabolde M, Guilloud-Bataille M, Feingold J, Besmond C. Association of variant alleles of mannose binding lectin with severity of pulmonary disease in cystic fibrosis: cohort study. BMJ. 1999;319(7218):1166-7.
21. Davies JC, Turner MW, Klein N; London MBL CF Study Group. Impaired pulmonary status in cystic fibrosis adults with two mutated MBL-2 alleles. Eur Respir J. 2004;24(5):798-804.
22. Garred P, Pressler T, Madsen HO, Frederiksen B, Svejgaard A, Høiby N, et al. Association of mannose-binding lectin gene heterogeneity with severity of lung disease and survival in cystic fibrosis. J Clin Invest. 1999;104(4):431-7.
23. Yarden J, Radojkovic D, De Boeck K, Macek M Jr, Zemkova D, Vavrova V, et al. Polymorphisms in the mannose binding lectin gene affect the cystic fibrosis pulmonary phenotype. J Med Genet. 2004;41(8):629-33.
24. Dorfman R, Sandford A, Taylor C, Huang B, Frangolias D, Wang Y, et al. Complex two-gene modulation of lung disease severity in children with cystic fibrosis. J Clin Invest. 2008;118(3):1040-9.
25. Arkwright PD, Laurie S, Super M, Pravica V, Schwarz MJ, Webb AK, et al. TGF-beta(1) genotype and accelerated decline in lung function of patients with cystic fibrosis. Thorax. 2000;55(6):459-62.
26. Wojnarowski C, Frischer T, Hofbauer E, Grabner C, Mosgoeller W, Eichler I, et al. Cytokine expression in bronchial biopsies of cystic fibrosis patients with and without acute exacerbation. Eur Respir J. 1999;14(5):1136-44.
27. Brazova J, Sismova K, Vavrova V, Bartosova J, Macek M Jr, Lauschman H, et al. Polymorphisms of TGF-beta1 in cystic fibrosis patients. Clin Immunol. 2006;121(3):350-7.
Sobre os autoresElisangela Jacinto de Faria
Doutora em Ciências Médicas. Faculdade de Ciências Médicas, Universidade Estadual de Campinas - Unicamp - Campinas (SP) Brasil.
Isabel Cristina Jacinto de Faria
Doutora em Ciências Médicas, Faculdade de Ciências Médicas. Universidade Estadual de Campinas - Unicamp - Campinas (SP) Brasil.
José Dirceu Ribeiro
Professor Livre-Docente. Departamento de Pediatria. Faculdade de Ciências Médicas, Universidade Estadual de Campinas - Unicamp - Campinas (SP) Brasil.
Antônio Fernando Ribeiro
Professor Livre-Docente em Gastropediatria. Faculdade de Ciências Médicas, Universidade Estadual de Campinas - Unicamp - Campinas (SP) Brasil.
Gabriel Hessel
Professor Livre-Docente em Gastroenterologia Pediátrica. Faculdade de Ciências Médicas, Universidade Estadual de Campinas - Unicamp - Campinas (SP) Brasil.
Carmen Sílvia Bertuzzo
Professora Livre-Docente. Departamento de Genética Médica, Faculdade de Ciências Médicas, Universidade Estadual de Campinas - Unicamp - Campinas (SP) Brasil.
* Trabalho realizado no Departamento de Genética Médica, Faculdade de Ciências Médicas, Universidade Estadual de Campinas - Unicamp - Campinas (SP) Brasil.
Endereço para correspondência: Elisangela Jacinto de Faria. Caixa Postal 6111, Cidade Universitária Zeferino Vaz, CEP 13083-970, Campinas, SP, Brasil.
Tel 55 19 3252-2603. E-mail: elliiss@yahoo.com.br
Suporte Financeiro: Nenhum.
Recebido para publicação em 20/8/2008. Aprovado, após revisão, em 17/9/2008.