ABSTRACT
Objective: To evaluate the effect of lung ischemic preconditioning (IPC) on normothermic ischemia/reperfusion (I/R) injury in a rat model,
quantifying the production of reactive oxygen species. Methods: Forty-seven male Wistar rats were randomized into four groups: control,
sham, I/R and IPC. Control group animals were anesthetized and killed by decapitation, after which pneumonectomy was performed and
the left lungs were stored in liquid nitrogen. Sham, IPC and I/R group rats were anesthetized, tracheostomized, ventilated, anticoagulated
and submitted to left thoracotomy with dissection of the left pulmonary artery for clamping. Sham group rats underwent dissection of the
left pulmonary artery, I/R group rats underwent 30 min of total hilar clamping, and IPC group rats underwent 5-min clamping of the left
pulmonary artery followed by 30 min of total hilar clamping. Lungs were reperfused for 90 min and ventilated with the same parameters,
with additional positive end-expiratory pressure of 1 cmH2O. Hemodynamic and blood gas values were obtained prior to thoracotomy,
prior to total hilar clamping, after 30 min of reperfusion and after 90 min of reperfusion. Lipid peroxidation was determined by measuring
levels of thiobarbituric acid reactive substances. Results: There were no significant differences among the groups in terms of the levels of
thiobarbituric acid reactive substances. Nor were there any significant differences among the sham, I/R and IPC groups in terms of arterial
oxygen tension, arterial carbon dioxide tension or hemodynamic values. Conclusions: In an in situ I/R rat model, 5-min IPC of the left
pulmonary artery does not attenuate I/R injury.
Keywords:
Ischemia; Reperfusion; Organ preservation; Reactive oxygen species.
RESUMO
Objetivo: Avaliar o efeito do pré-condicionamento isquêmico (PCI) em modelo de isquemia e reperfusão (I/R) pulmonar normotérmica em
ratos, quantificando a produção de espécies reativas do oxigênio. Métodos: Quarenta e sete ratos Wistar foram randomizados em quatro
grupos: controle, sham, I/R e PCI. Após anestesia, animais do grupo controle foram sacrificados por decapitação, pneumonectomizados,
e os pulmões esquerdos armazenados em nitrogênio líquido. Animais dos grupos sham, I/R e PCI foram anestesiados, traqueostomizados,
ventilados, anticoagulados e submetidos a uma toracotomia esquerda com dissecção da artéria pulmonar esquerda para clampeamento.
No grupo sham procedeu-se a dissecção da artéria pulmonar esquerda; no grupo I/R, clampeamento hilar total de 30 min e no grupo PCI,
clampeamento da artéria pulmonar esquerda por 5 min seguido por reperfusão de 10 min e um clampeamento hilar total de 30 min. Pulmões
foram reperfundidos por 90 min e ventilados com os mesmos parâmetros, acrescidos de pressão expiratória final positiva de 1 cmH2O. Foram
obtidas medidas hemodinâmicas e gasométricas antes da toracotomia, antes do clampeamento hilar total, aos 30 e 90 min de reperfusão. A
peroxidação lipídica foi estabelecida por meio da determinação das substâncias reativas ao ácido tiobarbitúrico. Resultados: A determinação
das substâncias reativas ao ácido tiobarbitúrico analisada nos grupos controle, sham, I/R, PCI não revelou diferenças significativas, o mesmo
ocorrendo com a pressão parcial arterial de oxigênio, pressão parcial arterial de gás carbônico e medidas hemodinâmicas entre os grupos
sham, I/R e PCI. Conclusões: O PCI de 5 min da artéria pulmonar esquerda em modelo de I/R in situ em ratos não atenua a lesão de I/R.
Palavras-chave:
Isquemia; Reperfusão; Preservação de órgãos; Espécies de oxigênio reativas.
IntroductionOxidative stress, characterized by the formation of reactive oxygen species (ROS), occurs in most pulmonary cells, most often through one of two mechanisms. One of these mechanisms involves the accumulation of hypoxanthine and the conversion of xanthine dehydrogenase into xanthine oxidase during hypoxia, followed by degradation of hypoxanthine into superoxide at the time of reoxygenation. The other mechanism depends on the reduced nicotinamide adenine dinucleotide phosphate system present in the endothelium and in the plasmatic membrane of neutrophils and macrophages. This mechanism is responsible for the reduction of oxygen into hydrogen peroxide and superoxide.(1)
In lung transplantation, the lesion induced by ischemia/reperfusion (I/R) of the graft, together with the low tolerance of this organ to ischemia, remains the principal cause of early morbidity and mortality in the postoperative period. This syndrome normally occurs within the first 72 h after transplantation and is characterized by the following conditions: increased pulmonary capillary permeability; pulmonary edema; hypoxemia; and greater pulmonary vascular resistance.(2)
Studies in animal models have focused on the prevention of I/R, as well as on the improvement of methods of lung preservation(2) through the use of lung hyperinflation,(3) hypothermic preservation,(4) lung preservation solutions,(5) vasodilation,(6) retrograde pulmonary perfusion,(7) ROS scavengers,(8) gene therapy,(9) liquid ventilation,(10) and ischemic preconditioning (IPC). The IPC technique consists of submitting an organ or tissue to a brief episode of I/R. This appears to confer protection against later, longer ischemic episodes and the resulting reperfusion injury. This strategy has been studied in many organs and tissues, such as the heart, kidney, liver, intestine, brain, skeletal muscle and lung,(11) in animal models as well as in clinical studies.(12) In most lung studies, IPC was achieved through total hilar clamping, which does not correspond to the clinical reality of transplantation, in which the pulmonary artery is isolated for clamping separately from the other hilar structures.
Based on these aspects, we tested the hypothesis that selective occlusion IPC would minimize I/R-related pulmonary injury in an experimental animal model.
MethodsThe project was approved by the Ethics in Research Committee (protocol no. 004084-5/6) of the Federal Foundation School of Medical Sciences of Porto Alegre. We used 47 male Wistar rats weighing between 250 g and 470 g. All of the animals were handled in accordance with the international standards regulating the use of laboratory animals.(13,14) The animals were randomized into four groups: control, sham, I/R (normothermic) and IPC. Figure 1 provides an overview of the procedures for each group:
° control group (n = 10): anesthetization and decapitation
° sham group (n = 12): anesthetization and 135 min of mechanical ventilation (MV) during which the pulmonary hilum was dissected (without clamping)
° I/R group (n = 13): anesthetization and 135 min of MV, during which there were 30 min of total hilar clamping, followed by 90 min of reperfusion
° IPC group (n = 12): anesthetization and 135 min of MV, during which the left pulmonary artery was clamped for 5 min (IPC), after which there were 10 min of reperfusion, followed by 30 min of total hilar clamping and 90 min of reperfusion
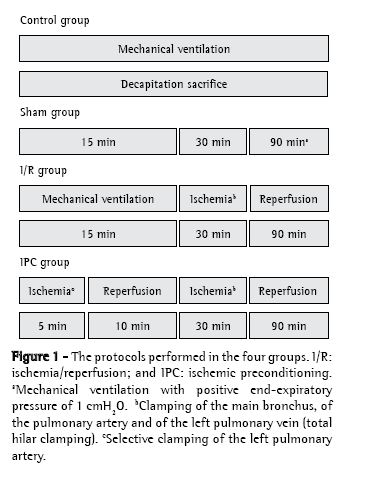
The animals were anesthetized with ketamine (100 mg/kg) and xylazine (15 mg/kg) via intraperitoneal injections, together with atropine (0.20 mg) via intramuscular injection at the time of induction, the anesthesia being maintained through administration of one-third doses of the drugs administered at 30-min intervals. Prior to being placed on MV, the animals received systemic heparin (1 mg/kg, parenteral) and were submitted to cervical tracheostomy with a plastic cannula (Abbocath® no. 14; Abbott Laboratories, Abbott Park, IL, USA). We employed a volumetric ventilator (Harvard Rodent Ventilator, model 683; Harvard Apparatus Co., Millis, MA, USA). The ventilator was set to a tidal volume of 10 mL/kg of body weight, a respiratory rate of 70-80 breaths/min, a fraction of inspired oxygen of 0.2 (on room air) and a positive end-expiratory pressure of 1 cmH2O only at the time of pulmonary reperfusion (the final 90 min of the procedure; Figure 1). At each total hilar clamping or selective arterial clamping, lung expansion was achieved through occlusion of the expiratory valve for three inspiratory cycles, in order to avoid atelectasis. Mean systemic arterial pressure was measured through cannulation of the right carotid artery (Sirecust 730; Siemens, Solna, Sweden), the same cannula being used in the collection of blood samples for the arterial blood gas analysis (ABL 5; Radiometer, Copenhagen, Denmark). Mean systemic arterial pressure and arterial blood gases were measured at various time points: before the thoracotomy (basal); before the 30-min period of ischemia (total hilar clamping) in the I/R and IPC groups; at min 15 of MV in the sham group; and at min 30 and 90 of reperfusion (before sacrifice).
A surgical microscope (Carl Zeiss, Oberkochen, Germany) was used in the dissection of the hilar structures and in the arterial cannulation. Following tracheotomy and cannulation of the right carotid artery, a left thoracotomy was performed in the fifth intercostal space, the pulmonary ligament was sectioned, and the left lung apex was caudally retracted with the aid of a cotton swab. Subsequently, the left pulmonary artery was isolated for selective clamping with the aid of a vascular microclip (Figure 1). After the 90-min period of reperfusion, the left lung was sectioned and stored at −80°C. The total time of MV to which the animals in the sham, I/R and IPC groups were submitted was 135 min (Figure 1).
The lungs stored at −80°C were defrosted to room temperature and homogenized with 9 mL of phosphate buffer (KCl 140 mM, phosphate 20 mM pH 7.4) per gram of tissue. Phenylmethylsulfonyl fluoride was added to the homogenate at a concentration of 100 nM in isopropanol and at a quantity of 10 μL/mL of added buffer, in addition to a protease inhibitor. Tissue samples were homogenized for 40 s at a temperature of 0-2°C in an Ultra-Turrax homogenizer (IKA Labortechnik, Staufen, Germany), after which they were centrifuged for 10 min at 3,000 rpm (1,110 × g) in a refrigerated centrifuge (Sorvall RC-5B refrigerated superspeed centrifuge; DuPont, Wilmington, DE, USA). The precipitate was discarded. The supernatant was removed and stored at −80°C for later analysis. The concentration of proteins in the homogenates was determined using a technique previously described.(15)
Levels of thiobarbituric acid reactive substancesThe levels of thiobarbituric acid reactive substances (TBARS) were determined. The tissue samples were placed in assay tubes, to which were added 0.75 mL of 10% trichloroacetic acid, 0.25 mL of the homogenate, 0.5 mL of 0.67% thiobarbituric acid and 25 mL of distilled water. Each tube was agitated and heated to 100°C, after which it was cooled and 1.5 mL of n-butyl alcohol were added. Subsequently, each was placed in an agitator (Biomatic, Porto Alegre, Brasil), agitated for 45 s and centrifuged for 10 min at 3,000 rpm (1,110 × g). Finally, the stained product was removed and read in a spectrophotometer (CARY 3E UV-Visible Spectrophotometer; Varian, Palo Alto, CA, USA) at a wavelength of 535 nm. The concentration of TBARS obtained is expressed as nmol/mg of protein.(16)
Statistical AnalysisData were compiled in a spreadsheet (MS Excel 7.0; Microsoft Corporation) and the statistical analysis was performed using the Statistical Package for the Social Sciences program, version 12.0 (SPSS Inc., Chicago, IL, USA). The groups were compared through analysis of variance. The level of statistical significance was 5%. Data are presented as mean and standard deviation.
ResultsThere were no statistically significant differences among the experimental groups (sham, I/R and IPC) regarding body weight (p = 0.822) or surgical time (p = 0.560), as well as, at any of the four time points analyzed, blood gas analysis findings, arterial oxygen tension (Figure 2) and arterial carbon dioxide tension (Figure 3). During the period of observation, there were gradual decreases in mean arterial pressure in all groups, although the differences among the experimental groups were not statistically significant at any of the four time points evaluated (Figure 4). In addition, there were no statistically significant differences among any of the groups in terms of the quantity of lipid peroxidation products, as assessed through determination of the levels of TBARS (p = 0.289; Figure 5).
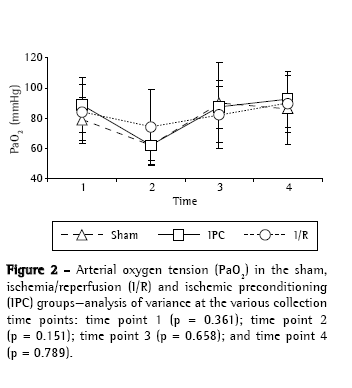
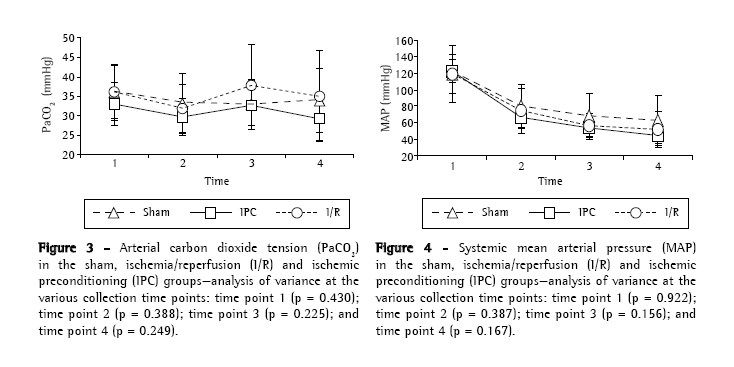
DiscussionLung injury due to I/R is a complex and multifactorial physiopathological phenomenon, which requires oxygen, together with the activation of vascular, humoral and cellular factors, for its genesis and maintenance. This condition remains the leading cause of morbidity and mortality in the postoperative period after lung transplantation. The clinical presentation ranges from mild hypoxemia with few infiltrates in the chest X-ray to a profile similar to that of acute respiratory distress syndrome.(1) The I/R can affect different organs and occur in many clinical situations such as surgical procedures used in conjunction with extracorporeal circulation, as well as thrombolytic therapy, aortic surgery, thromboembolectomy, hepatectomy and organ transplantation.(17) The principal factors responsible for this tissue damage are the oxygen free radicals, also known as ROS. Endothelial cells and leukocytes play important roles in this phenomenon, since ROS promote leukocyte activation and adherence to the microvascular endothelium, resulting in greater ROS production, as well as greater production of cytotoxic enzymes. In addition, hypoxia leads to greater endothelial permeability and the consequent edema.(1,18)
In most organs, ischemia is accompanied by tissue hypoxia until the reintroduction of oxygen during reperfusion, I/R therefore being equivalent to hypoxia-reoxygenation.(19) However, the lung is considered the only organ that can suffer ischemia without hypoxia, since the alveolar oxygen helps maintain the aerobic metabolism. Therefore, in the lung, the oxidative stress resulting from ischemia must be differentiated from that resulting from hypoxia.(1)
Hypoxia and the resulting anoxia cause a decrease in the quantity of intracellular adenosine triphosphate and an increase in the quantity of its degradation products, such as hypoxanthine, which promotes ROS production when the oxygen is reintroduced (through reperfusion or ventilation). During ischemia, this phenomenon can occur in the lungs if the alveolar oxygen tension drops below 7 mmHg.(3) The absence of pulmonary blood flow results in lipid peroxidation even in the presence of oxygen. The mechanism of oxidative stress is different from what occurs during hypoxia-reoxygenation because it is not associated with the decrease in adenosine triphosphate and can occur even during the period of cold ischemia in an organ stored for transplantation.(20)
The IPC technique has been studied as a means of minimizing I/R-related damage by promoting conditioning through metabolic, enzymatic and structural alterations, with consequent cellular protection against later reperfusion injury. This protective effect is due to what has been designated I/R tolerance.(11)
Since the results of various studies are contradictory, it is impossible to establish a relation between repeated episodes of IPC and a reduction in I/R-related tissue damage.(21,22) Du et al., using in a rat model of unilateral transplantation, were the first to report decreased ROS production in lungs submitted to IPC.(23) The effect that IPC has on I/R-related lung injury was also examined by other authors in various animal models: of isolated lung reperfusion(21,24,25); of in situ I/R(26-28); and of lung transplantation.(23,29)
Here, we used an experimental in situ model of I/R-related lung injury that was based on a model established in the literature and was used in a study previously conducted by our group.(30) In the present study, the IPC was performed using selective pulmonary arterial clamping, without occlusion of the bronchi or pulmonary veins. The IPC protocol used (5 min of ischemia followed by 10 min of reperfusion) was based on the observations of another group of authors,(27) who compared 5-min IPC with 10-min IPC in a canine model of in situ I/R injury. The authors found that the 5-min IPC resulted in better gas exchange and respiratory mechanics, as well as in lower expression of tumor necrosis factor-alpha in the bronchoalveolar lavage. In addition, Du et al. demonstrated that a 5-min IPC resulted in less I/R injury in a rat model of lung transplantation.(23) In the present study, the choice of a 90-min reperfusion time was based on the findings of another study.(31) The authors of that study employed an in situ I/R model and demonstrated, through chemiluminescence and continuous monitoring, that the production of superoxide radicals was uninterrupted and increased continuously until min 90 of reperfusion.
We found no statistical differences among the groups in terms of arterial blood gases or the degree of lipid peroxidation, as assessed by quantifying TBARS. These results differ from the findings of other studies,(23,27-29) in which IPC was found to minimize I/R injury. However, our findings corroborate those of another study.(24) An IPC-induced reduction in I/R injury has been demonstrated in two studies using an in situ model of I/R.(26,27) However, in those studies, the IPC was performed through total hilar clamping, different from the selective arterial clamping of the left pulmonary artery which we used. In lung transplantation models, the use of IPC as a form of lung preservation has been shown to present benefit, in rats(23) and in dogs.(29) However, in those studies, IPC was performed using total hilar clamping. In a model of isolated perfusion, another group of authors(24) observed the effect of IPC on lung compliance and on gas exchange by stopping the perfusion (for 5 or 10 min) with or without simultaneous respiratory arrest. It was concluded that there was a significant difference, in terms of lung compliance although not in terms of gas exchange, in the groups submitted to simultaneous stopping of the perfusion and ventilation. The results of that study are similar to those of the present study, in which IPC by selective clamping of the pulmonary artery did not reduce I/R injury in terms of blood gases or lipid peroxidation.
In conclusion, the results of the present study show that isolated 5-min IPC through selective occlusion of the pulmonary artery in a rat model of in situ I/R did not attenuate the I/R injury in terms of arterial blood gases or lipid peroxidation. Further studies should be carried out in order to clarify these findings.
References1. de Perrot M, Liu M, Waddell TK, Keshavjee S. Ischemia-reperfusion-induced lung injury. Am J Respir Crit Care Med. 2003;167(4):490-511.
2. de Perrot M, Keshavjee S. Lung preservation. Semin Thorac Cardiovasc Surg. 2004;16(4):300-8.
3. Puskas JD, Hirai T, Christie N, Mayer E, Slutsky AS, Patterson GA. Reliable thirty-hour lung preservation by donor lung hyperinflation. J Thorac Cardiovasc
Surg. 1992;104(4):1075-83.
4. Chien S, Zhang F, Niu W, Tseng MT, Gray L Jr. Comparison of university of wisconsin, euro-collins, low-potassium dextran, and krebs-henseleit solutions for hypothermic lung preservation. J Thorac Cardiovasc Surg. 2000;119(5):921-30.
5. Bertolotti A, Gómez C, Lascano E, Negroni J, Cuniberti L, Yannarelli G, et al. Effect of preservation solution on graft viability in single-lung transplantation from heart-beating donors in pigs. Transplant Proc. 2007;39(2):355-7.
6. Kohmann JC, Ruschel M, Madruga G, Castro M, Di Pietro D, Felicetti JC, et al. Efeito da prostaglandina E1 na viabilidade do pulmão de cadáver após transplante pulmonar unilateral em cães. J Pneumol 1994;20(Supl 3):145.
7. Kohmann JC, Silva UL, Madke G, Pilla ES, Felicetti JC, Camargo JJP, et al. Perfusão pulmonar anterógrada versus retrógrada na preservação pulmonar para transplante em modelo canino de viabilidade pulmonar pós-morte. J Pneumol. 1999;25(2):78-83.
8. Egan TM, Ulicny KS Jr, Lambert CJ Jr, Wilcox BR. Effect of a free radical scavenger on cadaver lung transplantation. Ann Thorac Surg. 1993;55(6):1453-9.
9. Itano H, Zhang W, Ritter JH, McCarthy TJ, Mohanakumar T, Patterson GA. Adenovirus-mediated gene transfer of human interleukin 10 ameliorates reperfusion injury of rat lung isografts. J Thorac Cardiovasc Surg. 2000;120(5):947-56.
10. Andrade CF, Martins LK, Tonietto TA, Koefender C, Anflor LC Jr, da Silva NB, et al. Partial liquid ventilation with perfluorodecalin following unilateral canine lung allotransplantation in non-heart-beating donors. J Heart Lung Transplant. 2004;23(2):242-51.
11. Pasupathy S, Homer-Vanniasinkam S. Ischaemic preconditioning protects against ischaemia/reperfusion injury: emerging concepts. Eur J Vasc Endovasc Surg. 2005;29(2):106-15.
12. Ambros JT, Herrero-Fresneda I, Borau OG, Boira JM. Ischemic preconditioning in solid organ transplantation: from experimental to clinics. Transpl Int. 2007;20(3):219-29.
13. Institute of Laboratory Animal Resources (U.S.). Guide for the Care and Use of Laboratory Animals. Washington, D.C.: National Academy Press, 1996. p. 1-35.
14. AVMA Panel on Euthanasia. American Veterinary Medical Association. 2000 Report of the AVMA Panel on Euthanasia. J Am Vet Med Assoc. 2001;218(5):669-96. Erratum in: J Am Vet Med Assoc 2001;218(12):1884.
15. Lowry OH, Rosebrough NJ, Farr AL, Randall RJ. Protein measurement with the Folin phenol reagent. J Biol Chem. 1951;193(1):265-75.
16. Buege JA, Aust SD. Microsomal lipid peroxidation. Methods Enzymol.
1978;52:302-10.
17. Carden DL, Granger DN. Pathophysiology of ischaemia-reperfusion injury. J Pathol. 2000;190(3):255-66.
18. Bast A, Haenen GR, Doelman CJ. Oxidants and antioxidants: state of the art. Am J Med. 1991;91(3C):2S-13S.
19. Dröge W. Free radicals in the physiological control of cell function. Physiol
Rev. 2002;82(1):47-95.
20. Eckenhoff RG, Dodia C, Tan Z, Fisher AB. Oxygen-dependent reperfusion injury in the isolated rat lung. J Appl Physiol. 1992;72(4):1454-60.
21. Gasparri RI, Jannis NC, Flameng WJ, Lerut TE, Van Raemdonck DE. Ischemic preconditioning enhances donor lung preservation in the rabbit. Eur J Cardiothorac Surg. 1999;16(6):639-46.
22. Iliodromitis EK, Kremastinos DT, Katritsis DG, Papadopoulos CC, Hearse DJ. Multiple cycles of preconditioning cause loss of protection in open-chest rabbits. J Mol Cell Cardiol. 1997;29(3):915-20.
23. Du ZY, Hicks M, Winlaw D, Spratt P, Macdonald P. Ischemic preconditioning enhances donor lung preservation in the rat. J Heart Lung Transplant. 1996;15(12):1258-67.
24. Featherstone RL, Chambers DJ, Kelly FJ. Ischemic preconditioning enhances recovery of isolated rat lungs after hypothermic preservation. Ann Thorac Surg. 2000;69(1):237-42.
25. Soncul H, Oz E, Kalaycioglu S. Role of ischemic preconditioning on ischemia-reperfusion injury of the lung. Chest. 1999;115(6):1672-7.
26. Li G, Chen S, Lu E, Hu T. Protective effects of ischemic preconditioning on
lung ischemia reperfusion injury: an in-vivo rabbit study. Thorac Cardiovasc Surg. 1999;47(1):38-41.
27. Friedrich I, Spillner J, Lu EX, Bartling B, Barnscheid M, Sablotzki A, et al. Ischemic pre-conditioning of 5 minutes but not of 10 minutes improves lung function after warm ischemia in a canine model. J Heart Lung Transplant.
2001;20(9):985-95.
28. Markart P, Schmidt R, Ruppert C, Höres C, Silber RE, Börgermann J, et al. Ischemic and endotoxin pre-conditioning reduce lung reperfusion injury-induced surfactant alterations. J Heart Lung Transplant. 2005;24(10):1680-9.
29. Li G, Chen S, Lou W, Lu E. Protective effects of ischemic preconditioning on donor lung in canine lung transplantation. Chest. 1998;113(5):1356-9.
30. Castro MA, Madruga GS, Lima e Silva U, Bock P, Klein AB, Felicetti JC, et al. Quantificaçäo dos produtos de peroxidaçäo lipídica em modelo experimental de injúria de reperfusäo pulmonar em ratos. J Pneumol. 1996;22(2):65-8.
31. Midorikawa J, Maehara K, Yaoita H, Watanabe T, Ohtani H, Ushiroda S, et al. Continuous observation of superoxide generation in an in-situ ischemia-reperfusion rat lung model. Jpn Circ J. 2001;65(3):207-12.
Study conducted at the Jean Carlo Kohmann Laboratory of Experimental Surgery of the Fundação Faculdade Federal de Ciências Médicas de Porto Alegre - FFFCMPA, Federal Foundation School of Medical Sciences of Porto Alegre - and at the Laboratory of Experimental Hepatology of the Universidade Federal do Rio Grande do Sul - UFRGS, Federal University of Rio Grande do Sul - Porto Alegre, Brazil.
1. Masters Student in the Postgraduate Program in Medical Sciences. Fundação Faculdade Federal de Ciências Médicas de Porto Alegre - FFFCMPA, Federal Foundation School of Medical Sciences of Porto Alegre - Porto Alegre, Brazil.
2. Medical Student. Fundação Faculdade Federal de Ciências Médicas de Porto Alegre - FFFCMPA, Federal Foundation School of Medical Sciences of Porto Alegre - Porto Alegre, Brazil.
3. Doctoral Student in the Postgraduate Program in Pulmonology. Universidade Federal do Rio Grande do Sul - UFRGS, Federal University of Rio Grande do Sul - Porto Alegre, Brazil.
4. Physical Therapist. Fundação Faculdade Federal de Ciências Médicas de Porto Alegre - FFFCMPA, Federal Foundation School of Medical Sciences of Porto Alegre - Porto Alegre, Brazil.
5. Full Professor of Human Physiology. Universidade Luterana do Brasil - ULBRA, Lutheran University of Brazil - Canoas, Brazil.
6. Thoracic Surgeon at the Porto Alegre Hospital de Clínicas. Universidade Federal do Rio Grande do Sul - UFRGS, Federal University of Rio Grande do Sul - Porto Alegre, Brazil.
7. Associate Professor in the Department of Surgery, Thoracic Surgery Sector. Fundação Faculdade Federal de Ciências Médicas de Porto Alegre - FFFCMPA, Federal Foundation School of Medical Sciences of Porto Alegre - Porto Alegre, Brazil.
Correspondence to: Paulo F. Guerreiro Cardoso. Pavilhão Pereira Filho, Santa Casa de Porto Alegre, Rua Prof. Annes Dias, 285, 1-PPF, CEP: 90020-090, Porto Alegre, RS, Brazil.
Tel: 55 51 3221-2232. E-mail: cardosop@gmail.com
Submitted: 9 October 2007. Accepted, after review: 26 November 2007.