ABSTRACT
Objective: Tuberculosis (TB) is an infectious disease caused by the bacillus Mycobacterium tuberculosis, which was recognized by the World Health Organization (WHO) as a global epidemic in 1993. TB is the leading infectious disease associated with silicosis, with studies showing an increased risk when compared to healthy individuals. We conducted an association study to evaluate the influence of polymorphisms in the ACE, FAM13A, FAS, FASLG, IL1RN, NOS2, TGFB1, and TNF genes on TB susceptibility. Methods: Nine polymorphisms were genotyped using Polymerase Chain Reaction (PCR) in a sample of 143 patients with silicosis in Rio de Janeiro (RJ), Brazil. Results: Seventy (49%) patients had a confirmed prior diagnosis of TB, of whom 25 (35.7%) had simple silicosis and 45 (64.3%) had complicated silicosis. The TG genotype of rs2609255 in FAM13A showed a protective effect against TB (OR=0.46; 95% CI: 0.22-0.98; p=0.040) compared to the GG genotype, and also when compared to the two combined homozygous genotypes (TT+GG) (OR=0.43; 95% CI: 0.20-0.90; p=0.024). Logistic regression analysis, including independent clinical variables, confirmed the protective effect of the TG genotype. Conclusion: This study suggests that the rs2609255 polymorphism in FAM13A may play a role in TB risk among patients with silicosis. Given the limited research on genetic polymorphisms and TB susceptibility in silicosis patients, further studies are needed to validate these findings.
Keywords:
silicosis, tuberculosis, genetic polymorphisms, genetic association studies, cytokines.
INTRODUCTION Tuberculosis (TB) is an infectious disease caused by the bacillus Mycobacterium tuberculosis (M. tuberculosis). Despite significant progress, it remains one of the most lethal infectious diseases worldwide.(1) Several conditions increase the risk of TB, including malnutrition, alcohol abuse, smoking, anemia, diabetes, anti-TNF drugs, HIV infection, and silicosis.(2–5)
Silicosis is a fibrotic lung disease caused by the inhalation of crystalline silica particles, and TB is its most commonly associated infectious disease. Studies have shown that individuals with silicosis have a higher risk of developing TB when compared to healthy individuals. (6) A recent meta-analysis by Ehrlich et al. (2021) further demonstrated that the severity of radiological manifestations of silicosis significantly increases the risk of TB.(6)
Tuberculosis is primarily transmitted through the air, but the mucociliary clearance mechanism plays a role in containing the bacillus.(7) Bacilli that evade this physical barrier reach the alveolar space, triggering the intense production of reactive oxygen species (ROS) and reactive nitrogen species (RNS), which cause direct damage to invading agents.(8) This oxidative stress environment results from increased levels of nitric oxide synthase (NOS2).(8,9) Additionally, it stimulates the release of several interleukins, including interleukin-1 (IL-1α, IL-1β), which promote fibroblast activation and collagen fiber deposition in the alveolar space, while the IL-1 receptor antagonist (IL-1Ra) inhibits their action.(10)
Bacilli that survive this intense oxidative environment are phagocytosed by alveolar macrophages (AM), triggering various inflammatory and anti-inflammatory pathways mediated by cells and multiple cytokines. (7) Several molecules are involved in these pathways, including tumor necrosis factor-alpha (TNFα), which is produced by activated AM and stimulates fibroblast recruitment and proliferation, in addition to serving as a ligand for apoptosis receptors.(9) TNFα also plays a crucial role in granuloma formation, a fundamental mechanism for containing M. tuberculosis. Activated AM also release anti-inflammatory cytokines, such as transforming growth factor beta-1 (TGFβ1), which downregulates proinflammatory cytokines and inhibits T-cell proliferation and activation, balancing the response between bacterial eradication and host survival.(11) Angiotensin-converting enzyme (ACE), released by epithelial cells and macrophages, is a biomarker of lung injury, and a gene polymorphism has recently been identified as a risk factor for TB.(12,13) AM apoptosis also contributes to the pathogenesis of TB and silicosis, with increased expression of cellular apoptosis receptors (FAS) and their ligands (FASLG and TNFα), promoting the recruitment of inflammatory cells.(9) Additionally, the interaction between AM and silica crystals elevates NOS2 levels, leading to increased nitric oxide production by macrophages and the generation of ROS and RNS, which cause extensive damage to cell membranes.(9,14)
The ability of macrophages and lymphocytes to migrate within interstitial compartments is essential for tissue surveillance and pathogen elimination. The Rho-GTPase family, a group of enzymes that bind and hydrolyze GTP, plays a fundamental role in cytoskeletal regulation and cell migration.(15) Several pathogenic bacteria secrete proteins into host cells, acting as activators or inhibitors of Rho-GTPases, thereby facilitating their pathogenesis. (16) M. tuberculosis also interferes with macrophage signaling pathways through GTP-binding proteins on Rho-GTPases, which are crucial for phagocytosis signal transduction.(16) The FAM13A isoform 1 protein contains a Rho GTPase-activating protein (RhoGAP) domain, suggesting its involvement in modulating Rho-GTPase activity.(17)
Despite the high risk of TB, some patients with silicosis do not develop this infectious complication, suggesting that genetic polymorphisms may influence susceptibility.(8,18,19) It is important to emphasize that silicosis is an irreversible and incurable disease, and that TB accelerates the progression of fibrosis in the lung parenchyma.(20)
Identifying biomarkers associated with a higher risk of TB in patients with silicosis is a valuable tool for disease management and follow-up. Therefore, the aim of the present study was to evaluate the influence of genetic polymorphisms in ACE (Ins/Del), FAM13A (rs2609255), FAS (rs2234767), FASLG (rs763110), IL1RN (rs419598 and rs2234663), NOS2 (rs2297518), TGFB1 (rs1800469), and TNF (rs1800629) on TB susceptibility in 143 Brazilian patients with silicosis. This is the first and largest Brazilian association study on genetic polymorphisms and tuberculosis in patients with silicosis.
METHODS Subjects This study included a total of 143 patients with silicosis, who were treated at the Antônio Pedro Hospital of the Federal Fluminense University, the Pedro Ernesto Hospital of the State University of Rio de Janeiro, and the National School of Public Health of the Oswaldo Cruz Foundation, all located in the State of Rio de Janeiro, Brazil. The study was approved by the Ethics Committees of these three institutions, and informed consent was obtained from all patients.
The diagnosis of silicosis was based on the occupational history of exposure to silica particles, in combination with radiological findings consistent with the disease, according to the International Classification of Radiographs of Pneumoconiosis (ILO). All chest radiographs were evaluated independently by three ILO-certified readers and classified as simple silicosis (opacities < 1.0 cm) or complicated silicosis (at least one opacity > 1.0 cm), following ILO criteria. The degree of agreement, measured using the Kappa coefficient, was 0.86. In cases of disagreement between readers regarding the classification of small and large opacities, the final result was determined by consensus among the three readers. The chest radiographs were obtained using Siemens AG equipment (model LX30; Erlangen, Germany) and a second device from VMI Tecnologias (LDM206-VM, MG, Brazil), applying the same radiological technique. At the time of diagnosis, all patients were removed from their workplaces. The patients included in this study were diagnosed with silicosis between 1969 and 2019, with a median diagnosis year of 2004.
The diagnosis of TB was confirmed through the association of clinical characteristics of the infectious disease with new radiological findings, in addition to confirmatory laboratory tests, such as a positive culture for M. tuberculosis, adenosine deaminase (ADA) levels, polymerase chain reaction (PCR), or histopathological findings of granulomatous disease with caseous necrosis. The diagnosis of TB occurred between 1975 and 2023, with a median diagnosis year of 2005.
Clinical characteristics The following social, clinical, and functional characteristics were evaluated: age (years), confirmed prior diagnosis of TB, occupational activity, silica exposure (SE; years), weekly working hours (WW; hours), total exposure time (TET; hours), exposure withdrawal (EW; years), smoking level (SL; pack-years), and use of personal protective equipment (PPE). The individual TET (in hours) was calculated by adjusting WW hours for each worked month (11/12; one-month vacation), which was then multiplied by the total number of working years. The EW (in years) was determined by subtracting the year of silicosis diagnosis (and work removal) from the year of sample collection
Laboratory procedures Genetic material was collected from cell samples obtained by mouthwash with 5 mL of saline solution (NaCl 0.5%) for 60 seconds. Individual samples were identified, and genomic DNA extraction was performed as previously described.(21)
Genotyping was carried out by PCR. IL1RN 2018T/C (rs419598), 86 bp variable number of tandem repeats (VNTR) (rs2234663), and ACE Ins/Del (rs4646994) were evaluated by standard PCR, as previously described.(13,22,23) For ACE rs4646994, the D allele, consisting of a 190-bp fragment, and the I allele, of a 490-bp fragment, were detected by electrophoresis of PCR products in a 2% agarose gel.
Genotypes of IL1RN rs2234663 (VNTR) were detected according to the PCR product sizes in a 2.5% agarose gel, which consisted of: IL1RN*1 (four repeats), 410 bp; IL1RN*2 (two repeats), 240 bp; IL1RN*3 (five repeats), 500 bp; IL1RN*4 (three repeats), 325 bp; and IL1RN*5 (six repeats), 595 bp. Restriction fragment length polymorphism (RFLP), using 1U of Msp I in a reaction following the manufacturer’s instructions (New England Biolabs), was used for genotyping IL1RN rs419598. Alleles were detected by electrophoresis in a 3% agarose gel, based on the following fragment sizes: 123 and 233 bp (C allele) and 356 bp (T allele).
Real-time polymerase chain reaction was performed using predesigned and validated TaqMan® assays (Thermo Fisher Scientific, Brazil) for genotyping FAM13A rs2609255 (C_15906608_10), FAS rs2234767 (-1377G/A; C_12123966_10), FASLG rs763110 (-844C/T; C_3175437_10), NOS2 rs2297518 (Ser608Leu; C_11889257_10), TGFB1 rs1800469 (-509C/T; C_8708473_10), and TNF rs1800629 (-308G/A; C_7514879_10). The samples were processed using the CFX96 real-time PCR system (Bio-Rad Laboratories, CA, USA), following the manufacturer’s instructions (Thermo Fisher Scientific, Brazil).
Statistical analyses Gene counting was performed to analyze allele frequencies. Deviations from Hardy-Weinberg equilibrium were evaluated using the χ2 test. The χ2 test or Fisher’s exact test was also used to perform association analyses between gene polymorphisms and TB. The t-test and Mann-Whitney test were used to analyze quantitative clinical characteristics with normal distribution and those with deviations from normal distribution, respectively, as determined by the Kolmogorov-Smirnov test. Values were presented as mean ± standard deviation (SD).
The odds ratio (OR) and 95% confidence interval (95% CI) for independent predictors of TB susceptibility were evaluated using multivariate logistic regression analysis. The logistic regression model included the following independent variables: polymorphisms significantly associated with TB, TET > 44,229 hours, EW in years, and silicosis severity (complicated silicosis). Statistical tests were performed using SPSS version 20.0 software (SPSS Inc., Chicago, IL, USA). A p-value < 0.05 was considered significant.
RESULTS Clinical and radiological characteristics Among the 143 patients, 66 (46%) worked in sandblasting, the most prevalent occupation in our sample. Other occupations included marble working (21 patients, 15%), hammer drilling (18 patients, 12%), civil construction (17 patients, 12%), and various other activities (tilemaker, sandblasting manager, welder, painter, ceramicist), which accounted for 21 patients (15%).
Seventy patients (49%) had a confirmed prior diagnosis of TB, including 25 (35.7%) with simple silicosis and 45 (64.3%) with complicated silicosis (p=0.321). Among these 70 TB cases, 57 (81%) had pulmonary tuberculosis, 11 (16%) had pleural tuberculosis, and 2 (3%) had lymph node tuberculosis. The clinical parameters and diagnostic procedures are shown in Table 1.
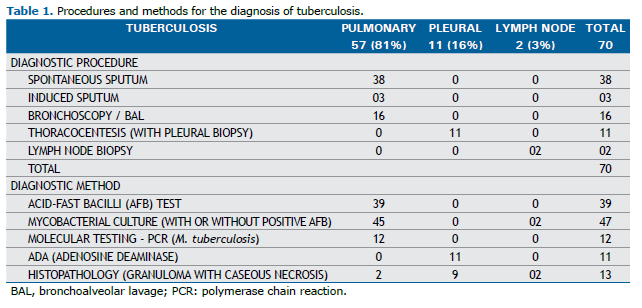
Regarding the radiographic classification of patients without a confirmed prior diagnosis of TB according to the ILO 2022 criteria, 28 patients were categorized as having simple chronic silicosis, with categories 1 (1/1 and 1/2) and 2 (2/1 and 2/2) being the most prevalent in this group, accounting for 68% of cases. In this group, 37 patients presented with large opacities and were classified as follows: 13 (35%) as category A, 17 (46%) as category B, and 7 (19%) as category C. Among patients with a confirmed prior diagnosis of TB, as classified by the ILO 2022, 25 were categorized as having simple chronic silicosis, with categories 2 (2/1 and 2/2) and 3 (3/2 to 3/3) being the most prevalent, together comprising 73% of cases. In this group, 42 patients presented with large opacities and were classified as follows: 11 (26%) as category A, 19 (45%) as category B, and 12 (29%) as category C. Other clinical and demographic characteristics are presented in Tables 2 and 3.
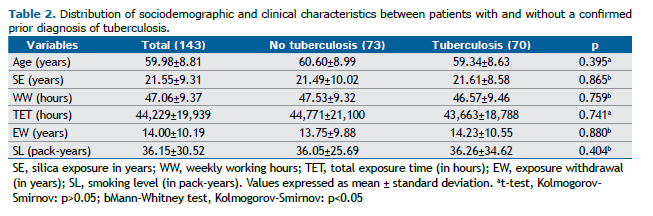
No statistically significant differences were observed in silica exposure (SE) (p=0.865), weekly working hours (WW) (p=0.759), total exposure time (TET, hours) (p=0.741), exposure withdrawal (EW) (p=0.880), or smoking level (SL) (p=0.404) between patients with and without a confirmed prior diagnosis of TB.
Association analyses The genotype distribution of ACE (Ins/Del), FAM13A (rs2609255), FAS (rs2234767), FASLG (rs763110), IL1RN (rs419598 and rs2234663), NOS2 (rs2297518), TGFB1 (rs1800469), and TNF (rs1800629) polymorphisms was in equilibrium according to the Hardy-Weinberg principle.
A summary of genotype and allele distribution in the total sample and among individuals with a confirmed prior diagnosis of TB is provided in Table 4. A statistically significant association was observed between rs2609255 in FAM13A and TB. The TG genotype exhibited a protective effect against TB (OR=0.46; 95% CI: 0.22–0.98; p=0.040). Additionally, the analysis revealed that the FAM13A TG genotype was also a predictor of protection against TB (OR=0.43; 95% CI: 0.20–0.90; p=0.024) when compared to the combined homozygous genotypes (TT+GG) (Table 4). No significant associations were found for the remaining polymorphisms.
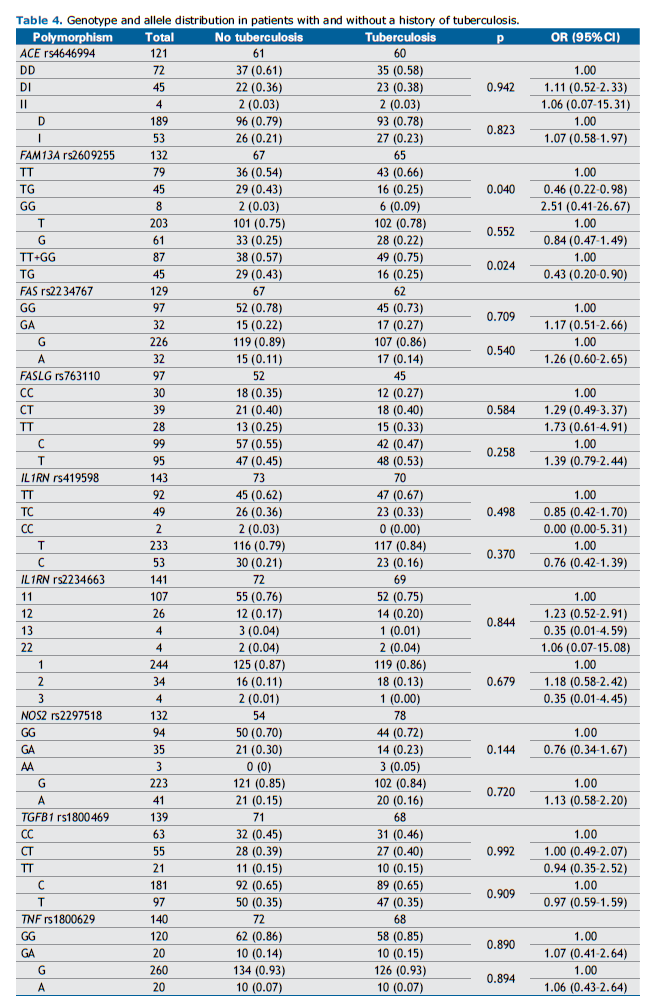
The multiple logistic regression model, including the potential independent risk variables for TB susceptibility, is presented in Table 5. After adjusting for other risk factors, rs2609255 in FAM13A was the only independent variable significantly associated with TB. The FAM13A TG genotype exhibited a protective effect against TB susceptibility (OR=0.32; 95% CI: 0.13–0.75; p=0.009), while no effect was observed for EW, TET, or silicosis severity (complicated silicosis).
DISCUSSION The respiratory system has mechanisms that prevent the entry of various agents, such as particulate inorganic material, viruses, bacteria, and mycobacteria. Additionally, several leukocytes that compose the immune system contribute to the defense process, with AM being the most important.(24,25)
Macrophages phagocytose bacilli and, after breaking them down, present their main antigens to lymphocytes. This antigen presentation triggers a cascade of biological events in lymphocytes, including activation, clonal expansion, and cytokine secretion, which in turn activate other macrophages. AM also serve as a reservoir for harboring bacilli, allowing intracellular multiplication. The bacilli are ultimately eliminated from the AM interior through apoptosis.(26)
The ability of leukocytes to migrate through interstitial compartments is essential for tissue surveillance and pathogen elimination. Each stage of this migration relies on the dynamic regulation of the cytoskeleton. The Rho-GTPase families play a fundamental role in cytoskeleton regulation, thereby ensuring adequate cell migration.(15) Rho-GTPases transmit signals from cell surface receptors and regulate the actin cytoskeleton and microtubules, controlling cell shape, polarity, mobility, and adhesion.(17)
Changes in Rho-GTPases have been described in lung diseases such as asthma, COPD, and acute lung injury,(17) and are also associated with infectious diseases.(16) Salmonella typhimurium, for example, secretes the SptP protein, which functions as a GTPase-activating protein (GAP) on Rho-GTPases, influencing the host cell.(27)
Tuberculosis is also associated with Rho-GTPases. (16) Sun et al. (2010) described how the mycobacterial nucleoside diphosphate kinase protein (Ndk) exhibited activity on the GTPase-activating protein, inhibiting phagosome maturation and thereby promoting the survival of mycobacteria within macrophages.(28) The same authors also demonstrated that the NdK protein was associated with a reduction in nitric oxide production mediated by NOS2, interfering with apoptosis dependent on reactive oxygen species and affecting the activity of Rho-GTPases.(29)
The FAM13A isoform 1 protein contains a RhoGAP domain, suggesting its involvement in modulating the activity of Rho-GTPases.(17) The FAM13A (family with sequence similarity 13, member A) is expressed in the airways, particularly in type II epithelial cells and macrophages of human lungs, and its coding gene is located on chromosome 4q22.(17,30,31) Two variants of FAM13A have been identified in human lung tissue, with 24 exons and 17 exons, respectively.(17) Corvol et al. (2014) described how genetic variation in FAM13A affects Rho-GTPase activation and is associated with cellular pathways, though its exact biological function remains poorly understood.(17)
In this study, we observed an association between the rs2609255 polymorphism of the FAM13A gene and TB in patients with silicosis. The TG genotype showed a protective effect against TB susceptibility in these patients. Since the FAM13A protein contains a RhoGAP domain, the rs2609255 polymorphism in FAM13A could act as a modulator of Rho-GTPase activity, thereby promoting host protection mechanisms against Mycobacterium tuberculosis bacilli. However, no functional study of this polymorphism has been conducted to date. Other studies have also shown associations between polymorphisms in FAM13A and various lung diseases, such as idiopathic pulmonary fibrosis,(31,32) asthma,(33) chronic obstructive pulmonary disease (COPD),(34) cystic fibrosis,(35) and also increased susceptibility to silicosis.(30) This increased susceptibility to silicosis could be explained by other mechanisms. A recent study revealed that FAM13A can activate the Wnt pathway, a network of protein interactions that regulates several cellular functions, including cell differentiation, migration, and proliferation, and controls the protein stability of β-catenin.(36) The Wnt/β-catenin pathway has been reported to play a role in the development and progression of silicosis, with β-catenin acting as a key regulator of this process.(37,38) Moreover, the Wnt/β-catenin pathway has been shown to be significantly activated in lung tissue from patients with lung fibrosis, and the aberrant activation of this pathway may induce increased fibroblast migration.(39) In addition, the pharmacological and genetic inhibition of the Wnt /β-catenin pathway may attenuate, or even reverse, lung fibrosis.(40)
No associations were observed with the other polymorphisms evaluated in this study. This lack of association may be attributed to certain study limitations, such as the inability to analyze all pathways of TB pathogenesis and the small sample size. Further studies are needed to investigate the associations between genetic polymorphisms and the pathophysiological mechanisms of TB in patients with silicosis. Given the limited number of studies correlating genetic polymorphisms with TB susceptibility in patients with silicosis, additional research is required to confirm our findings.
ACKNOWLEDGMENTS Financial support was provided by the National Council for Scientific and Technological Development (CNPq, Brazil; No. 402244/2016-8 and 310147/2023-9), the Rio de Janeiro State Research Support Foundation (FAPERJ, Brazil; No. E-26/210.293/2024), and the Pro-Rectorate of Research, Graduate Studies, and Innovation of the Federal Fluminense University (Proppi/PDI/UFF).
AUTHOR CONTRIBUTIONS Study conception or design: FBK and ASFN; sample collection: MCSC, ASFN, KCRS, VMB, HC, PC, WC, and FBK; data acquisition, analysis, and interpretation: MCSC, KCRS, VMB, CBMN, and FBK; drafting the manuscript or revising it critically for important intellectual content: MCSC, JMR, and FBK; final approval of the manuscript: MCSC, ASFN, KCRS, VBM, JMR, HC, PC, WC, CBMN, and FBK.
REFERENCES 1. Cortez AO, Melo AC, Neves LO, Resende KA, Camargos P. Tuberculosis in Brazil: one country, multiple realities. J Bras Pneumol. 2021;47(2):e20200119. https://doi.org/10.36416/1806-3756/e20200119.
2. Lönnroth K, Jaramillo E, Williams BG, Dye C, Raviglione M. Drivers of tuberculosis epidemics: the role of risk factors and social determinants. Soc Sci Med. 2009;68(12):2240–6. https://doi.org/10.1016/j.socscimed.2009.03.041.
3. Gelaw Y, Getaneh Z, Melku M. Anemia as a risk factor for tuberculosis: a systematic review and meta-analysis. Environ Health Prev Med. 2021;26(1):13. https://doi.org/10.1186/s12199-020-00931-z.
4. Silva DR, Muñoz-Torrico M, Duarte R, Galvão T, Bonini EH, Arbex FF, et al. Risk factors for tuberculosis: diabetes, smoking, alcohol use, and the use of other drugs. J Bras Pneumol. 2018;44(2):145–52. https://doi.org/10.1590/s1806-37562017000000443.
5. Jamshidi P, Danaei B, Arbabi M, Mohammadzadeh B, Khelghati F, Akbari Aghababa A, et al. Silicosis and tuberculosis: A systematic review and meta-analysis. Pulmonology. 2025;30(1):2416791. https://doi.org/10.1016/j.pulmoe.2023.05.001.
6. Ehrlich R, Akugizibwe P, Siegfried N, Rees D. The association between silica exposure, silicosis and tuberculosis: a systematic review and meta-analysis. BMC Public Health. 2021;21(1):953. https://doi.org/10.1186/s12889-021-10711-1.
7. O’Garra A, Redford PS, McNab FW, Bloom CI, Wilkinson RJ, Berry MP. The immune response in tuberculosis. Annu Rev Immunol. 2013;31:475–527. https://doi.org/10.1146/annurev-immunol-032712-095939.
8. Qu Y, Tang Y, Cao D, Wu F, Liu J, Lu G, et al. Genetic polymorphisms in alveolar macrophage response-related genes, and risk of silicosis and pulmonary tuberculosis in Chinese iron miners. Int J Hyg Environ Health. 2007;210(6):679–89. https://doi.org/10.1016/j.ijheh.2006.11.010.
9. Lopes-Pacheco M, Bandeira E, Morales MM. Cell-Based Therapy for Silicosis. Stem Cells Int. 2016;2016:5091838. https://doi.org/10.1155/2016/5091838.
10. Zhou Y, Kang Y, Zhang Z, Liu J. IL-1RA +2018 polymorphism and the susceptivity to pneumoconiosis: a Meta-analysis. Int J Clin Exp Med. 2014;7(8):2204–8. PMID: 25232408; PMCID: PMC4161568.
11. Flynn JL, Chan J, Lin PL. Macrophages and control of granulomatous inflammation in tuberculosis. Mucosal Immunol. 2011;4(3):271–8. https://doi.org/10.1038/mi.2011.14.
12. Porchera DCRF, Leal DFVB, Braga ACO, Pinto PDC, Santana da Silva MN, Bezerra Santos LC, et al. Association of the rs4646994 in ACE gene with susceptibility to tuberculosis in a region of the Brazilian Amazon. Transl Med Commun. 2022;7(1):10. https://doi.org/10.1186/s41231-022-00116-6.
13. Mohebbi I, Abdi Rad I, Bagheri M. Association of angiotensin-1-converting enzyme gene variations with silicosis predisposition. Inhal Toxicol. 2010;22(13):1110–5. https://doi.org/10.3109/08958378.2010.526654.
14. Castranova V. Signaling pathways controlling the production of inflammatory mediators in response to crystalline silica exposure: role of reactive oxygen/nitrogen species. Free Radic Biol Med. 2004;37(7):916–25. https://doi.org/10.1016/j.freeradbiomed.2004.05.032.
15. Biro M, Munoz MA, Weninger W. Targeting Rho-GTPases in immune cell migration and inflammation. Br J Pharmacol. 2014;171(24):5491-506. https://doi.org/10.1111/bph.12658.
16. Chopra P, Koduri H, Singh R, Koul A, Ghildiyal M, Sharma K, et al. Nucleoside diphosphate kinase of Mycobacterium tuberculosis acts as GTPase-activating protein for Rho-GTPases. FEBS Lett. 2004;571(1–3):212–6. https://doi.org/10.1016/j.febslet.2004.06.073.
17. Corvol H, Hodges CA, Drumm ML, Guillot L. Moving beyond genetics: is FAM13A a major biological contributor in lung physiology and chronic lung diseases? J Med Genet. 2014;51(10):646–9. https://doi.org/10.1136/jmedgenet-2014-102525.
18. Qu YB, Tang YX, Zhang ZB, Zhu R, Liu J, Gu SY, et al. [Relationship between single nucleotide polymorphisms of NRAMP1 gene and susceptibility to pulmonary tuberculosis in workers exposed to silica dusts]. Zhonghua Lao Dong Wei Sheng Zhi Ye Bing Za Zhi. 2006;24(9):531–3. Chinese. PMID: 17034726.
19. Salum KCR, de Castro MCS, Moreira VB, Nani ASF, Kohlrausch FB. Interleukin 1α and 1β gene variations are associated with tuberculosis in silica exposed subjects. Am J Ind Med. 2020;63(1):74–84. https://doi.org/10.1002/ajim.23066.
20. Lee HS, Phoon WH, Ng TP. Radiological progression and its predictive risk factors in silicosis. Occup Environ Med. 2001;58(7):467–71. https://doi.org/10.1136/oem.58.7.467.
21. Aidar M, Line SR. A simple and cost-effective protocol for DNA isolation from buccal epithelial cells. Braz Dent J. 2007;18(2):148–52. https://doi.org/10.1590/s0103-64402007000200012.
22. Kim SK, Kang SW, Chung JH, Lee JS, Park HK, Yoon KL, et al. Coding single-nucleotide polymorphisms of interleukin-1 gene cluster are not associated with Kawasaki disease in the Korean population. Pediatr Cardiol. 2011;32(4):381–5. https://doi.org/10.1007/s00246-010-9858-7.
23. Rad IA, Mohebbi I, Bagheri M. Molecular Evaluation of the IFN γ +874, TNF α -308, and IL-1Ra VNTR Sequences in Silicosis. Maedica (Bucur). 2012;7(1):20–4. PMID: 23118815; PMCID: PMC3484791.
24. Lohmann-Matthes ML, Steinmüller C, Franke-Ullmann G. Pulmonary macrophages. Eur Respir J. 1994;7(9):1678–89. PMID: 7995399.
25. Zhang P, Summer WR, Bagby GJ, Nelson S. Innate immunity and pulmonary host defense. Immunol Rev. 2000;173:39–51. https://doi.org/10.1034/j.1600-065x.2000.917306.x.
26. Flynn JL, Chan J. Immunology of tuberculosis. Annu Rev Immunol. 2001;19:93–129. https://doi.org/10.1146/annurev.immunol.19.1.93.
27. Fu Y, Galán JE. A salmonella protein antagonizes Rac-1 and Cdc42 to mediate host-cell recovery after bacterial invasion. Nature. 1999;401(6750):293–7. https://doi.org/10.1038/45829.
28. Sun J, Wang X, Lau A, Liao TY, Bucci C, Hmama Z. Mycobacterial nucleoside diphosphate kinase blocks phagosome maturation in murine RAW 264.7 macrophages. PLoS One. 2010;5(1):e8769. https://doi.org/10.1371/journal.pone.0008769.
29. Sun J, Singh V, Lau A, Stokes RW, Obregón-Henao A, Orme IM, et al. Mycobacterium tuberculosis nucleoside diphosphate kinase inactivates small GTPases leading to evasion of innate immunity. PLoS Pathog. 2013;9(7):e1003499. https://doi.org/10.1371/journal.ppat.1003499.
30. Wang W, Yu Y, Wu S, Sang L, Wang X, Qiu A, et al. The rs2609255 polymorphism in the FAM13A gene is reproducibly associated with silicosis susceptibility in a Chinese population. Gene. 2018;661:196–201. https://doi.org/10.1016/j.gene.2018.03.098.
31. Hirano C, Ohshimo S, Horimasu Y, Iwamoto H, Fujitaka K, Hamada H, et al. FAM13A polymorphism as a prognostic factor in patients with idiopathic pulmonary fibrosis. Respir Med. 2017;123:105–9. https://doi.org/10.1016/j.rmed.2016.12.007.
32. Hu Y, Li Z, Ren Y, Dai H. Association of family sequence similarity gene 13A gene polymorphism and interstitial lung disease susceptibility: A systematic review and meta-analysis. Mol Genet Genomic Med. 2023;11(11):e2279. https://doi.org/10.1002/mgg3.2279.
33. Deng CW, Zhang XX, Lin JH, Huang LF, Qu YL, Bai C. Association between Genetic Variants of Transforming Growth Factor-β1 and Susceptibility of Pneumoconiosis: A Meta-analysis. Chin Med J (Engl). 2017;130(3):357–64. https://doi.org/10.4103/0366-6999.198917.
34. Cho MH, Boutaoui N, Klanderman BJ, Sylvia JS, Ziniti JP, Hersh CP, et al. Variants in FAM13A are associated with chronic obstructive pulmonary disease. Nat Genet. 2010;42(3):200–2. https://doi.org/10.1038/ng.535.
35. Wright JM, Merlo CA, Reynolds JB, Zeitlin PL, Garcia JG, Guggino WB, et al. Respiratory epithelial gene expression in patients with mild and severe cystic fibrosis lung disease. Am J Respir Cell Mol Biol. 2006;35(3):327–36. https://doi.org/10.1165/rcmb.2005-0359OC.
36. Jin Z, Chung JW, Mei W, Strack S, He C, Lau GW, et al. Regulation of nuclear-cytoplasmic shuttling and function of Family with sequence similarity 13, member A (Fam13a), by B56-containing PP2As and Akt. Mol Biol Cell. 2015;26(6):1160–73. https://doi.org/10.1091/mbc.E14-08-1276.
37. Wang X, Xu K, Yang XY, Liu J, Zeng Q, Wang FS. Upregulated miR-29c suppresses silica-induced lung fibrosis through the Wnt/β-catenin pathway in mice. Hum Exp Toxicol. 2018;37(9):944–52. https://doi.org/10.1177/0960327117741750.
38. Dai W, Liu F, Li C, Lu Y, Lu X, Du S, et al. Blockade of Wnt/β-Catenin Pathway Aggravated Silica-Induced Lung Inflammation through Tregs Regulation on Th Immune Responses. Mediators Inflamm. 2016;2016:6235614. https://doi.org/10.1155/2016/6235614.
39. Chilosi M, Poletti V, Zamò A, Lestani M, Montagna L, Piccoli P, et al. Aberrant Wnt/beta-catenin pathway activation in idiopathic pulmonary fibrosis. Am J Pathol. 2003;162(5):1495–502. https://doi.org/10.1016/s0002-9440(10)64282-4.
40. Lam AP, Herazo-Maya JD, Sennello JA, Flozak AS, Russell S, Mutlu GM, et al. Wnt coreceptor Lrp5 is a driver of idiopathic pulmonary fibrosis. Am J Respir Crit Care Med. 2014;190(2):185–95. https://doi.org/10.1164/rccm.201401-0079OC.